Electrochemistry, Electrolysis, different electrolyzer
Introduction
Electrochemistry is the branch of chemistry that deals with the study of the relationships between electrical and chemical processes. It is concerned with the behavior of charged particles and their interactions with one another in the presence of an electric field.
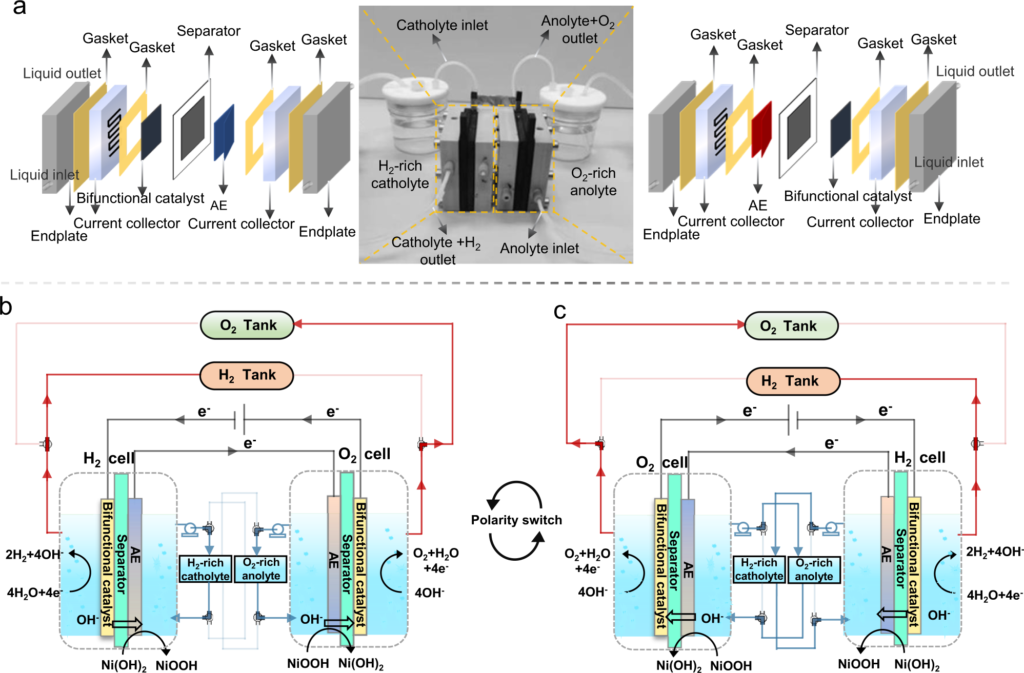
One important aspect of electrochemistry is electrolysis, which is the process by which an electric current is used to cause a chemical reaction. Electrolysis involves passing an electric current through an electrolyte, which is a solution or a molten substance that conducts electricity.
During electrolysis, positive ions move towards the negative electrode (cathode), where they gain electrons and are reduced, while negative ions move towards the positive electrode (anode), where they lose electrons and are oxidized. The overall reaction depends on the electrolyte and the electrodes used.
Electrolysis is used for a variety of purposes, such as electroplating, metal refining, and the production of chemicals. One of the most important applications of electrolysis is the production of hydrogen, which is a clean and renewable fuel.
To produce hydrogen through electrolysis, an electrolyzer is used. An electrolyzer is a device that uses an electric current to split water into hydrogen and oxygen. There are several types of electrolyzers, including alkaline electrolyzers, polymer electrolyte membrane (PEM) electrolyzers, and solid oxide electrolyzers.
Alkaline electrolyzers are the most mature technology and have been used for many years. They use an alkaline solution as the electrolyte and operate at high temperatures and pressures. Alkaline electrolyzers are efficient and reliable, but they are also bulky and require regular maintenance.
PEM electrolyzers, on the other hand, use a polymer electrolyte membrane as the electrolyte and operate at low temperatures and pressures. They are compact and efficient, but they also require expensive materials and can be sensitive to impurities in the feedstock.
Solid oxide electrolyzers use a solid oxide electrolyte and operate at high temperatures. They are highly efficient and can tolerate impurities in the feedstock, but they also require expensive materials and can be less durable than other types of electrolyzers.
In conclusion, electrochemistry and electrolysis play important roles in many industrial and technological applications, including the production of hydrogen through electrolyzers. Alkaline, PEM, and solid oxide electrolyzers are three different types of electrolyzers that are used to produce hydrogen, each with their own advantages and disadvantages. Continued research and development in this field will be essential to improving the efficiency, cost-effectiveness, and reliability of electrolysis and electrolyzers, and to advancing the use of hydrogen as a clean and renewable fuel.
Electrochemistry is a discipline of chemistry that focuses on the transformations that occur in a matter as a result of the passage of an electric current and the subsequent conversion of chemical energy to electrical energy and vice versa. Cells and batteries are two primary examples of technologies that are capable of converting chemical energy into electrical energy.
Basic of Electrochemistry
Electrochemistry is the branch of chemistry that deals with the study of chemical reactions that involve the transfer of electrons between substances. It is concerned with the relationship between electrical energy and chemical reactions.
Electrochemical reactions involve the movement of electrons between two substances, one acting as an electron donor (oxidation) and the other as an electron acceptor (reduction). This transfer of electrons is facilitated by a conductor, such as a metal wire, and occurs through an electrochemical cell.
An electrochemical cell consists of two electrodes, a cathode and an anode, that are connected by a conductor and separated by an electrolyte, which is a substance that conducts electricity. The cathode is the electrode where reduction occurs, and the anode is the electrode where oxidation occurs. The electrolyte serves as a medium for the transfer of charged particles, such as ions.
During an electrochemical reaction, electrons are transferred from the anode to the cathode, and the flow of electrons generates an electric current. The chemical reaction is said to be spontaneous if it can produce an electric current, and non-spontaneous if an external source of energy is needed to produce the current.
One of the most common applications of electrochemistry is in batteries. Batteries are electrochemical cells that store and release electrical energy through a chemical reaction. The reaction occurs between the electrodes and the electrolyte, and the flow of electrons generates an electric current.
Electrochemistry also has many other applications, such as in electroplating, where a metal coating is deposited on a surface by passing an electric current through a solution containing the metal ions, and in corrosion prevention, where a metal surface is protected from corrosion by connecting it to a more active metal that will corrode in its place.
Overall, electrochemistry plays an important role in many industrial and technological applications, and its understanding is essential for the development of new technologies and processes.
Oxidation and reduction reactions, or redox processes, refer to chemical reactions involving the transfer of electrons. These reactions provide electricity in a battery or galvanic cell when correctly arranged. Each half-cell of a galvanic cell consists of an electrode and an electrolyte solution.
A redox reaction consists of two Half Reactions: an oxidation reaction and a reduction reaction.
Each half reaction may be configured as a Half Cell, and the combination of two Half Cells creates a basic battery. A galvanic cell may be considered a battery, however, batteries consist of many galvanic cells connected in series to produce larger voltages.
Oxidation of a species (atom, ion, or molecule) results in the loss of electron(s), while reduction results in the acquisition of electron(s) (s). The Oxidation States are defined by certain standards, and the oxidation of an atom produces a rise in its oxidation state. In contrast, reduction decreases a substance’s oxidation state.
Balancing Redox Equations is a difficult undertaking, however, using half-reactions is a very effective method.
The driving factor behind chemical reactions is energy. Electrochemical potentials (E), or cell electromagnetic fields (EMF), of galvanic cells are connected to the energies of oxidation and reduction processes. The standard reduction potentials for half cells are realistic comparison values, since a set of norms has been adhered to. The energy (also known as Gibb’s free energy G) is the reaction’s electric work. Therefore,
The difference between Gibb’s free energy (G) between products and reactants is equal to the product of the charge n F and the potential difference ΔEo. Since n electrons are transported in the equation, the charge involved in units of moles is n F (F is the Faraday constant of 96485 C).
The equilibrium constant K is related to ΔG∘ ,
For this reaction,
aA + bB
Corresponding Nernst Equation:

Electrolysis
Electrolysis is a process in which an electric current is used to drive a non-spontaneous chemical reaction. It involves the use of an electrolytic cell, which is a device that contains an electrolyte (a solution or a molten substance that conducts electricity) and two electrodes (an anode and a cathode).
During electrolysis, a voltage is applied across the two electrodes, causing electrons to flow from the anode to the cathode. The anode undergoes oxidation (loses electrons) while the cathode undergoes reduction (gains electrons), resulting in the formation of new chemical species at each electrode.
The overall reaction that occurs during electrolysis depends on the electrolyte and the electrodes used. For example, if the electrolyte is water (H2O) and the electrodes are made of platinum, the reaction that occurs at the anode is the oxidation of water to form oxygen gas (O2) and hydrogen ions (H+), while at the cathode, the reduction of water to form hydrogen gas (H2) and hydroxide ions (OH–) occurs.
Electrolysis has many important industrial applications. For example, it is used in the production of chlorine and sodium hydroxide by the electrolysis of brine (sodium chloride solution). During this process, chlorine gas is produced at the anode while hydrogen gas and sodium hydroxide are produced at the cathode.
Another important application of electrolysis is in the production of aluminum from bauxite ore. In this process, the aluminum oxide in the ore is dissolved in molten cryolite (an electrolyte) and electrolyzed using carbon electrodes. The reduction of aluminum ions at the cathode produces molten aluminum metal, while carbon dioxide gas is produced at the anode.
Electrolysis also has potential applications in energy storage and conversion. For example, it can be used in the production of hydrogen gas by the electrolysis of water, which can then be used as a fuel for vehicles or in fuel cells to produce electricity. The development of more efficient and cost-effective electrolysis technologies is an active area of research and development in the field of renewable energy.
In conclusion, electrolysis is a powerful tool for driving non-spontaneous chemical reactions and has many important industrial applications. Its use in energy storage and conversion also holds promise for a more sustainable and clean energy future.
Electrons get their driving power from chemical reactions that take place in batteries or galvanic cells. These reactions give the electrons the ability to battle through burdens. This is the process that takes place when chemical energy is converted into electric energy. Electrolysis may be performed either in solutions or in salts that have been melted (liquid). Fluidity is required of the medium since the atoms and ions need to be able to move about in it physically. Just like the reactants in a galvanic cell, the products may exist in any of the three states: solid, liquid, or gas.
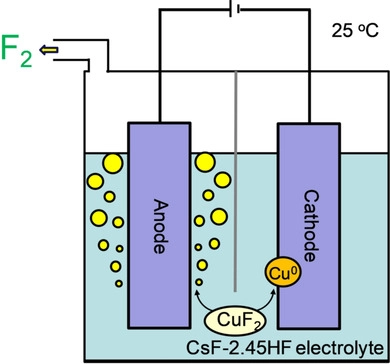
Molten Salts Electrolysis:
Electrolysis is a method that entails forcing electrons through a chemical cell, which ultimately results in a chemical reaction being produced. The positive charge will typically draw electrons to itself, and the electrode that is responsible for supplying those electrons is referred to as the cathode since reduction occurs on that electrode.
According to the definition, reduction takes happens only at the cathode. The following processes take place at the cathode and the anode during the electrolysis of molten salt, also known as NaCl:
Cathode (reduction): Na++e−→Na
Anode (oxidation): 2Cl−→Cl2+2e−
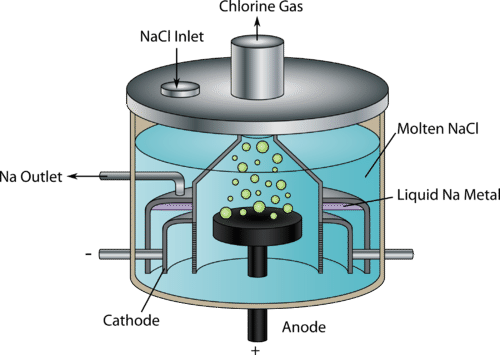
Water Electrolysis:
Electrolysis is an exciting possibility for the creation of carbon-free hydrogen from renewable and nuclear sources of energy. The process of electrolysis involves the use of electricity in order to separate hydrogen and oxygen from water. A device referred to as an electrolyzer is where this process takes occurs. Electrolyzers can range in size from small, appliance-size equipment that is well-suited for small-scale distributed hydrogen production to large-scale, central production facilities that could be directly tied to renewable or other non-greenhouse gas-emitting forms of electricity production. Small, appliance-size electrolyzers are well-suited for small-scale hydrogen production on a distributed scale.
Water electrolysis is the process of splitting water molecules into hydrogen and oxygen gas using an electric current. It is a well-established and versatile technology that has numerous applications in industry, research, and renewable energy.
Water electrolysis involves the use of an electrolytic cell that contains an electrolyte solution, two electrodes (an anode and a cathode), and a power source. When a voltage is applied across the two electrodes, an electric current flows through the electrolyte, causing water molecules to be split into hydrogen ions (H+) and hydroxide ions (OH-). The hydrogen ions are attracted to the cathode, where they are reduced to form hydrogen gas (H2), while the oxygen ions are attracted to the anode, where they are oxidized to form oxygen gas (O2).
There are two main types of water electrolysis: alkaline electrolysis and proton exchange membrane (PEM) electrolysis. Alkaline electrolysis is the most established and widely used technology, while PEM electrolysis is a newer technology that has gained increasing attention in recent years.
Alkaline electrolysis uses a strong alkali electrolyte solution, such as potassium hydroxide (KOH), sodium hydroxide (NaOH), or lithium hydroxide (LiOH). The electrolyte solution is typically housed in a tank and circulated through the electrolytic cell. The anode and cathode are made of different metals, such as nickel or platinum, and are separated by a porous diaphragm that prevents the mixing of the gases produced.
PEM electrolysis, on the other hand, uses a solid polymer electrolyte membrane that selectively allows the transport of protons (H+) between the anode and cathode. The electrodes are typically made of platinum or other noble metals, and the electrolyte membrane is typically made of a perfluorinated polymer such as Nafion. PEM electrolysis has the advantage of being more efficient and requiring less energy than alkaline electrolysis, but it is also more expensive and requires more careful maintenance.
Water electrolysis has numerous applications in industry and research. One of the most important applications is in the production of hydrogen gas, which is used as a feedstock for the production of ammonia, methanol, and other chemicals. Hydrogen gas can also be used as a fuel for vehicles, either through combustion in an internal combustion engine or through conversion in a fuel cell to produce electricity.
Another important application of water electrolysis is in the production of oxygen gas, which is used in medical applications, such as respiratory support for patients with lung disease, and in industrial processes, such as metal cutting and welding.
Water electrolysis also has potential applications in the storage and conversion of renewable energy. One of the main challenges of renewable energy is its intermittency, which makes it difficult to match supply and demand. Water electrolysis can be used to store excess renewable energy in the form of hydrogen gas, which can then be used as a fuel when renewable energy supply is low. The use of hydrogen gas as a fuel can also help to reduce greenhouse gas emissions and increase energy security.
Despite its numerous applications and potential benefits, water electrolysis still faces several challenges. One of the main challenges is the high cost of the technology, which is primarily due to the cost of the electrodes and the power source. Improvements in materials science and manufacturing processes are needed to reduce the cost of the technology.
Another challenge is the efficiency of the electrolysis process, which is still relatively low compared to other energy conversion technologies. Improvements in the design of electrolytic cells and the development of new electrode materials are needed to increase the efficiency of the process.
In conclusion, water electrolysis is a versatile and important technology that has numerous applications in industry, research, and renewable energy.
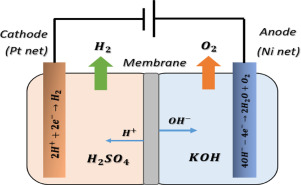
Working Mechanism:
Electrolyzers, which are very similar to fuel cells, are made up of an anode and a cathode that are separated by an electrolyte. Different types of electrolyzers each have their own unique methods of operation, which are mostly determined by the electrolyte material that is used and the ionic species that are conducted by it.
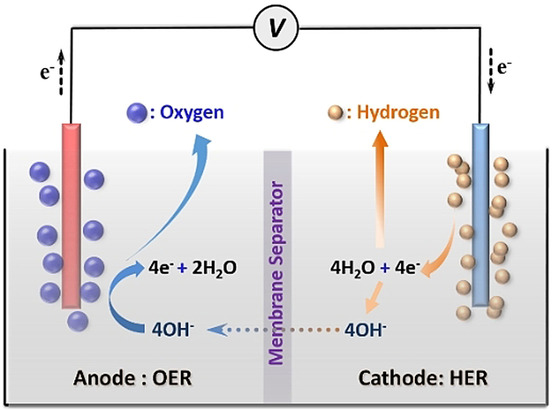
Polymer Electrolyte Membrane Electrolyzers:
The electrolyte of a polymer electrolyte membrane (PEM) electrolyzer is a solid specialized plastic material. This kind of electrolyzer is used to produce electricity.
At the anode, water undergoes a reaction that results in the formation of oxygen and positively charged hydrogen ions (protons).
The electrons go through an external circuit, and the hydrogen ions pass over the PEM in a selective manner so that they may arrive at the cathode.
At the cathode, hydrogen ions mix with electrons that have been brought in from the outside circuit to produce hydrogen gas.
Anode Reaction: 2H2O → O2 + 4H+ + 4e–
The reaction at the cathode is as follows: 2H+ + 2e– →H2
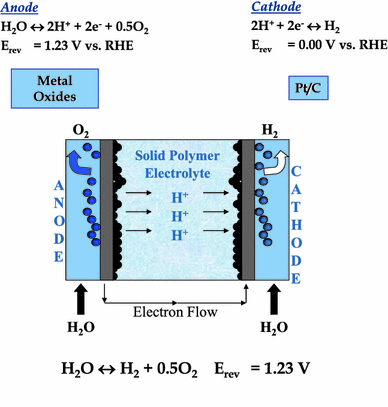
Polymer electrolyte membrane (PEM) electrolyzers are a type of water electrolyzer that uses a solid polymer electrolyte membrane to selectively transport protons between the anode and cathode. PEM electrolysis has gained increasing attention in recent years due to its higher efficiency and lower energy consumption compared to traditional alkaline electrolysis.
The basic operating principle of a PEM electrolyzer is similar to that of an alkaline electrolyzer. The electrolytic cell consists of an anode and a cathode separated by a solid polymer electrolyte membrane. Water is fed into the cell and an electric current is applied across the electrodes. The electric current causes the water molecules to dissociate into hydrogen ions and oxygen gas.
The hydrogen ions are transported across the membrane to the cathode, where they are reduced to form hydrogen gas. The oxygen gas is produced at the anode through the oxidation of water. Unlike in alkaline electrolysis, the electrolyte in PEM electrolysis is a solid polymer that allows for the selective transport of protons but prevents the mixing of hydrogen and oxygen gases.
PEM electrolysis has several advantages over alkaline electrolysis. One of the main advantages is its higher efficiency, which can be attributed to the use of the proton-selective membrane. The solid polymer electrolyte membrane reduces the voltage required for water electrolysis, resulting in lower energy consumption and higher efficiency. In addition, PEM electrolysis has a faster response time and can operate at higher pressures, making it suitable for certain industrial applications.
PEM electrolysis also has several other advantages over alkaline electrolysis, including its ability to operate at lower temperatures and its ability to produce high-purity hydrogen gas without the need for a gas purification system. The high-purity hydrogen gas produced by PEM electrolysis is suitable for use in fuel cells and other applications that require high-purity hydrogen.
However, there are also several challenges associated with PEM electrolysis. One of the main challenges is the cost of the technology. PEM electrolyzers are generally more expensive than alkaline electrolyzers, primarily due to the cost of the membrane and the electrodes. Improvements in materials science and manufacturing processes are needed to reduce the cost of PEM electrolysis.
Another challenge is the durability of the membrane. The membrane can be degraded over time by exposure to water, high temperatures, and other factors. Improvements in membrane materials and design are needed to increase the durability of the membrane and reduce the need for frequent replacement.
In conclusion, polymer electrolyte membrane (PEM) electrolysis is a promising technology for the production of hydrogen gas and other applications. It has several advantages over traditional alkaline electrolysis, including higher efficiency, lower energy consumption, and the ability to produce high-purity hydrogen gas. However, there are also challenges associated with the technology, including its cost and durability. Continued research and development in materials science and manufacturing processes are needed to overcome these challenges and make PEM electrolysis a more cost-effective and sustainable technology.
Alkaline Electrolyzers
Hydrogen is produced on the cathode side of alkaline electrolyzers by the transfer of hydroxide ions (OH-) through the electrolyte from the cathode to the anode. This process is known as the alkaline electrolysis reaction. Electrolyzers that use a liquid alkaline solution of sodium or potassium hydroxide as the electrolyte have been commercially available for many years. These solutions may either be used to generate sodium hydroxide or potassium hydroxide. On a smaller scale, more recent methods that make use of solid alkaline exchange membranes (AEM) as the electrolyte are showing encouraging results.
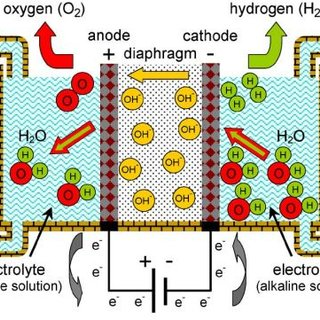
An alkaline electrolyzer is a type of electrochemical cell that uses an alkaline solution, typically potassium hydroxide (KOH) or sodium hydroxide (NaOH), as the electrolyte. It operates by passing an electric current through water to split it into its constituent elements, hydrogen and oxygen. The overall reaction is:
2H2O → 2H2 + O2
The anode in an alkaline electrolyzer is typically made of a metal such as nickel or nickel-plated steel, while the cathode is usually made of a porous material such as nickel or stainless steel. When a direct current is applied, water molecules are oxidized at the anode to form oxygen gas and positively charged hydrogen ions (protons). The protons then migrate through the electrolyte to the cathode, where they are reduced to form hydrogen gas.
One advantage of alkaline electrolyzers is that they can operate at high current densities, which allows for high production rates of hydrogen gas. They are also relatively simple and inexpensive to manufacture. However, they require a high-purity source of water to avoid contamination of the electrolyte, and the alkaline solution can be corrosive to certain materials. Additionally, alkaline electrolyzers typically require higher voltages to operate than other types of electrolyzers, which can result in higher energy costs.
Difference between by alkaline electrolyzers and Polymer Electrolyte Membrane Electrolyzers
Here’s a table summarizing some key differences between alkaline electrolyzers and polymer electrolyte membrane (PEM) electrolyzers:
Feature | Alkaline Electrolyzers | PEM Electrolyzers |
---|---|---|
Electrolyte | Alkaline solution (e.g. KOH or NaOH) | Polymer membrane (e.g. Nafion) |
Operating temperature | Relatively high (typically 60-90°C) | Relatively low (typically 50-80°C) |
Operating pressure | Relatively low (typically 1-30 bar) | Relatively high (typically 10-30 bar) |
Efficiency | Moderate to high (70-80%) | High (up to 80-90%) |
Production rate | High | Moderate to high |
Durability | Relatively high (10-15 years) | Relatively low (5-10 years) |
Cost | Relatively low | Relatively high |
Some additional notes:
- PEM electrolyzers use a polymer membrane to separate the anode and cathode compartments, while alkaline electrolyzers use an alkaline solution as the electrolyte.
- Alkaline electrolyzers typically operate at higher temperatures and lower pressures than PEM electrolyzers.
- PEM electrolyzers generally have higher efficiency and better durability than alkaline electrolyzers, but they are typically more expensive to manufacture.
- Both types of electrolyzers can produce hydrogen at a high rate, but alkaline electrolyzers may be better suited for large-scale production due to their lower cost and high production rate.
Solid Oxide Electrolyzers
Solid oxide electrolyzers create hydrogen in a somewhat different fashion than other types of electrolyzers. These electrolyzers employ a solid ceramic substance as the electrolyte, which, when heated to high temperatures, preferentially conducts negatively charged oxygen ions (O2-). When electrons from the external circuit mix with electrons already present in the steam at the cathode, they produce hydrogen gas and positively charged oxygen ions.
The oxygen ions go through the solid ceramic membrane and then undergo a reaction at the anode, which results in the production of oxygen gas and electrons for the external circuit.
PEM electrolyzers operate at temperatures ranging from 70°C to 90°C, while commercial alkaline electrolyzers typically operate at temperatures of less than 100°C. Solid oxide electrolyzers are required to operate at temperatures high enough for the solid oxide membranes to function properly. These temperatures range from approximately 700°C to 800°C. There is hope for decreasing the operating temperature to between 500 and 600 degrees Celsius with the development of new lab-scale solid oxide electrolyzers that are based on proton-conducting ceramic electrolytes. Solid oxide electrolyzers are able to make efficient use of the heat that is available at these increased temperatures (which may come from a variety of sources, including nuclear energy), which allows them to reduce the amount of electrical energy that is required to create hydrogen from water.
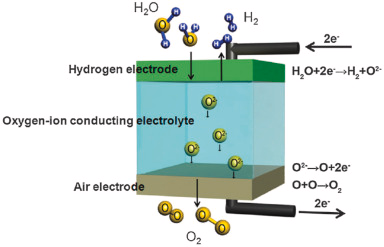