Introduction
Lithium-ion batteries are rechargeable energy storage devices utilized in a variety of applications, such as consumer electronics, electric vehicles, and renewable energy systems. The components include a cathode, anode, separator, and electrolyte. During charge, lithium ions travel from the cathode to the anode, whereas during discharging, they move in the opposite direction. This allows the battery to store and release electrical energy. Lithium-ion batteries have a high energy density, an extended cycle life, and a low rate of self-discharge. However, they have drawbacks such as a short lifespan and the possibility of thermal runaway. Lithium-ion batteries have revolutionized portable power and remain the favored option for many contemporary applications.
Lithium-ion batteries (LIBs) are rechargeable batteries that use lithium ions as the active material to store and release energy. They have become the preferred energy storage technology in many applications, including portable electronics, electric vehicles, and grid energy storage systems.
The raw materials of lithium-ion batteries include several key components, each with its own unique properties and specifications. These components include the anode, cathode, electrolyte, and separator.
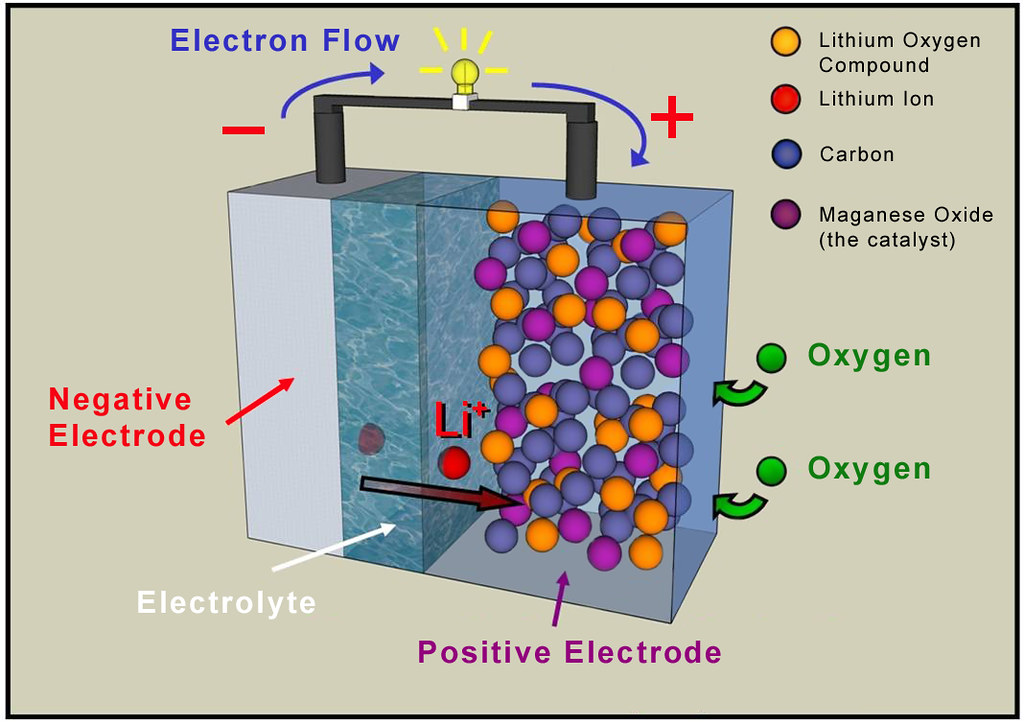
Anode
The anode is the negative electrode of a lithium-ion battery, and it is typically made of graphite. Graphite is a form of carbon that is known for its stability, conductivity, and ability to intercalate lithium ions. Intercalation is the process of inserting lithium ions between the layers of graphite, which allows the battery to store and release energy.
An anode is one of the key components of a lithium-ion battery. It is the electrode where the oxidation reaction takes place during the battery’s discharge cycle. The anode materials used in lithium-ion batteries play a crucial role in determining the battery’s performance characteristics, including energy density, power density, and cycle life. The selection of anode materials is thus critical to the overall design and performance of the battery.
The most common anode material used in lithium-ion batteries is graphite, a form of carbon. Graphite has excellent electrical conductivity, high natural abundance, and low cost. It also has a high specific surface area, allowing it to store a large amount of lithium ions. Graphite is used in the anode because it is able to intercalate lithium ions between its layers, a process known as intercalation. During charging, lithium ions are extracted from the cathode and move through the electrolyte to the anode, where they are stored in the graphite layers. During discharging, the lithium ions move back to the cathode, releasing energy as they do so.
While graphite is the most commonly used anode material, there are other materials being researched for their potential as anodes in lithium-ion batteries. One promising candidate is silicon. Silicon has a much higher theoretical capacity than graphite, meaning it can store more lithium ions per unit mass. This would lead to higher energy density and longer cycle life. However, silicon has several drawbacks, including low electrical conductivity, a tendency to fracture during cycling, and a large volume expansion during lithiation and delithiation. These issues are currently being addressed through various methods, including nanostructuring, coating, and alloying with other metals.
Another potential anode material is lithium titanate (Li4Ti5O12). Unlike graphite, lithium titanate has a low voltage profile, which means it can be charged and discharged at a high rate without causing thermal runaway. It also has a long cycle life and excellent stability. However, lithium titanate has a lower energy density than graphite, meaning it would require a larger battery to achieve the same energy storage capacity.
Other anode materials being researched include metal oxides, metal sulfides, and carbon nanotubes. These materials have varying properties and potential advantages but also come with their own set of challenges that need to be addressed before they can be widely adopted.
In addition, Lithium-ion batteries use various materials as the anode (negative electrode) to store and release lithium ions during charge and discharge cycles. Here is a list of commonly used anode materials in lithium-ion batteries:
- Graphite: Graphite is the most widely used anode material in commercial lithium-ion batteries. It has excellent stability and a high capacity to intercalate lithium ions, providing good cycling performance and long-term stability.
- Silicon: Silicon has a high theoretical capacity for lithium storage, making it an attractive candidate for high-energy-density batteries. However, silicon undergoes significant volume expansion and contraction during charge and discharge, which can lead to mechanical stress and capacity loss. Researchers are actively working on developing silicon-based anodes with enhanced stability and cycling performance.
- Lithium titanate (Li4Ti5O12): Lithium titanate is a spinel structure material that offers excellent stability and safety. It has a relatively low capacity compared to graphite or silicon, but it provides excellent cycling performance, fast charging capabilities, and long cycle life. Lithium titanate anodes are often used in applications where safety and durability are crucial.
- Tin-based materials: Tin-based materials, such as tin oxide (SnO2) and tin composite alloys, have high theoretical capacities and are being explored as potential anode materials. However, similar to silicon, tin-based materials experience significant volume changes during cycling, which can lead to mechanical degradation. Research efforts are focused on addressing these challenges to improve their practical viability.
- Lithium metal: While not commonly used as an anode material in commercial lithium-ion batteries, pure lithium metal has the highest theoretical capacity. However, lithium metal anodes face significant challenges related to dendrite formation, which can cause safety hazards and reduce the battery’s cycle life. Researchers are actively working on developing strategies to mitigate dendrite formation and improve the stability of lithium metal anodes.
It’s important to note that the choice of anode material depends on various factors, including the specific application, desired energy density, cycle life requirements, and safety considerations. Ongoing research and development efforts aim to improve anode materials and develop new materials to enhance the performance, safety, and energy density of lithium-ion batteries.
In summary, the anode materials used in lithium-ion batteries play a crucial role in determining the battery’s performance characteristics. Graphite is the most commonly used anode material due to its excellent conductivity, natural abundance, and low cost. Other materials, such as silicon and lithium titanate, are being researched for their potential to improve energy density, power density, and cycle life.
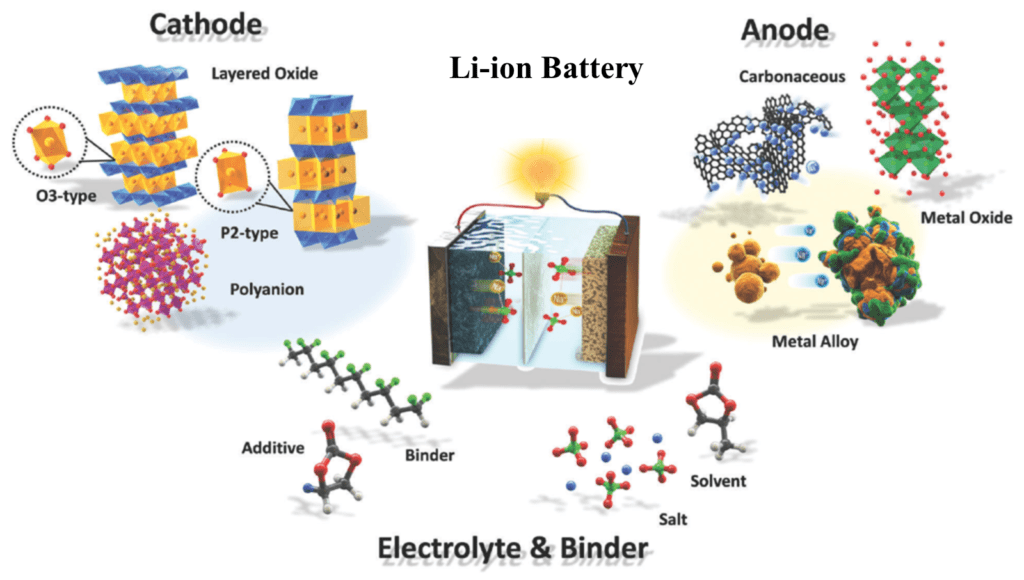
Cathode, Anode Materials, and Electrolytes in Li-ion Battery
Cathode
The cathode is the positive electrode of a lithium-ion battery, and it is typically made of metal oxide. The metal oxide is chosen based on its specific properties, including its ability to intercalate lithium ions and its stability. Common cathode materials include lithium cobalt oxide (LCO), lithium nickel manganese cobalt oxide (NMC), and lithium iron phosphate (LFP).
In a lithium-ion battery, the cathode is the positive electrode that receives the electrons from the external circuit during discharge, while the anode is the negative electrode that donates electrons during discharge. The cathode materials are crucial to the performance of lithium-ion batteries, as they are responsible for storing and releasing lithium ions during charge and discharge cycles. In this article, we will discuss the different types of cathode materials used in lithium-ion batteries and their properties.
- Lithium Cobalt Oxide (LCO) – LCO is one of the most commonly used cathode materials in lithium-ion batteries due to its high energy density. It is composed of cobalt oxide and lithium ions, and has a theoretical capacity of 274 mAh/g. However, LCO has some disadvantages, such as poor thermal stability, low cycle life, and high cost.
- Lithium Manganese Oxide (LMO) – LMO is a cathode material composed of lithium, manganese, and oxygen. It has a high thermal stability and a long cycle life, making it a popular choice for power tools and electric vehicles. However, LMO has a lower energy density compared to other cathode materials.
- Lithium Iron Phosphate (LFP) – LFP is a cathode material composed of lithium, iron, and phosphate. It has a high thermal stability, long cycle life, and low cost. LFP also has a lower risk of thermal runaway and is less prone to overheating, making it a safer option for applications where safety is a concern. However, LFP has a lower energy density compared to other cathode materials.
- Lithium Nickel Manganese Cobalt Oxide (NMC) – NMC is a cathode material composed of lithium, nickel, manganese, and cobalt. It has a high energy density, good thermal stability, and a long cycle life. NMC is used in many consumer electronic devices and electric vehicles. However, NMC has some disadvantages, such as a high cost and a lower thermal stability compared to LMO.
- Lithium Nickel Cobalt Aluminum Oxide (NCA) – NCA is a cathode material composed of lithium, nickel, cobalt, and aluminum. It has a high energy density and a long cycle life, making it a popular choice for electric vehicles. However, NCA has some disadvantages, such as a high cost and low thermal stability.
- Lithium Titanate (LTO) – LTO is a cathode material composed of lithium and titanium. It has a high thermal stability and a long cycle life, making it a popular choice for stationary energy storage systems. LTO also has a higher power density compared to other cathode materials. However, LTO has a lower energy density compared to other cathode materials, which limits its use in certain applications.
In conclusion, cathode materials play a crucial role in the performance of lithium-ion batteries. Each type of cathode material has its own advantages and disadvantages, and the choice of material depends on the specific application requirements. Researchers are continually exploring new cathode materials to improve the performance and safety of lithium-ion batteries.
Electrolyte
The electrolyte is the substance that facilitates the movement of ions between the anode and cathode of a lithium-ion battery. The electrolyte is typically a liquid or gel substance that contains lithium salts, such as lithium hexafluorophosphate (LiPF6), dissolved in an organic solvent, such as ethylene carbonate or diethyl carbonate. The choice of an electrolyte depends on factors such as the desired operating voltage, temperature range, and safety requirements.
In a lithium-ion battery, the electrolyte is a critical component that enables the movement of ions between the anode and the cathode during the charge and discharge cycles. The electrolyte acts as a mediator that carries lithium ions between the electrodes, allowing for the flow of electric current. The performance and safety of the battery are highly dependent on the characteristics and properties of the electrolyte.
Electrolytes used in lithium-ion batteries are typically liquid, solid, or gel-like, and can be classified based on their chemical composition and properties. Some of the most common types of electrolyte materials used in lithium-ion batteries include:
- Organic Carbonate-Based Electrolytes: These electrolytes are typically composed of a mixture of organic solvents, such as ethylene carbonate (EC) and dimethyl carbonate (DMC), and lithium salts, such as lithium hexafluorophosphate (LiPF6) or lithium perchlorate (LiClO4). These materials are commonly used in commercial lithium-ion batteries due to their high conductivity, wide voltage range, and low cost.
- Polymer-Based Electrolytes: These electrolytes are made up of a polymer matrix, such as polyethylene oxide (PEO), that is mixed with lithium salts to form a solid-state electrolyte. Polymer-based electrolytes have the advantage of being more stable and safer than liquid electrolytes, and they can also be fabricated into flexible and thin film batteries.
- Ceramic-Based Electrolytes: Ceramic electrolytes, such as lithium aluminum titanium phosphate (LATP) and lithium lanthanum titanate (LLTO), have high ionic conductivity and good thermal stability. They are also non-flammable and non-volatile, making them a safer alternative to liquid electrolytes. Ceramic electrolytes are commonly used in high-performance and high-temperature applications, such as electric vehicles and grid storage systems.
- Ionic Liquid-Based Electrolytes: Ionic liquids are a type of salt that has a liquid state at room temperature. They are highly conductive and have low volatility, making them a promising alternative to traditional liquid electrolytes. However, they are still relatively expensive and have limited availability.
The choice of electrolyte material depends on several factors, including the type of application, performance requirements, and safety considerations. In addition to conductivity and stability, other properties such as viscosity, density, and chemical compatibility with other battery components are also important considerations in selecting an appropriate electrolyte material.
Overall, the development of new and advanced electrolyte materials is critical to improving the performance, safety, and sustainability of lithium-ion batteries, as well as enabling the development of new and emerging applications such as wearable electronics and electric vehicles.
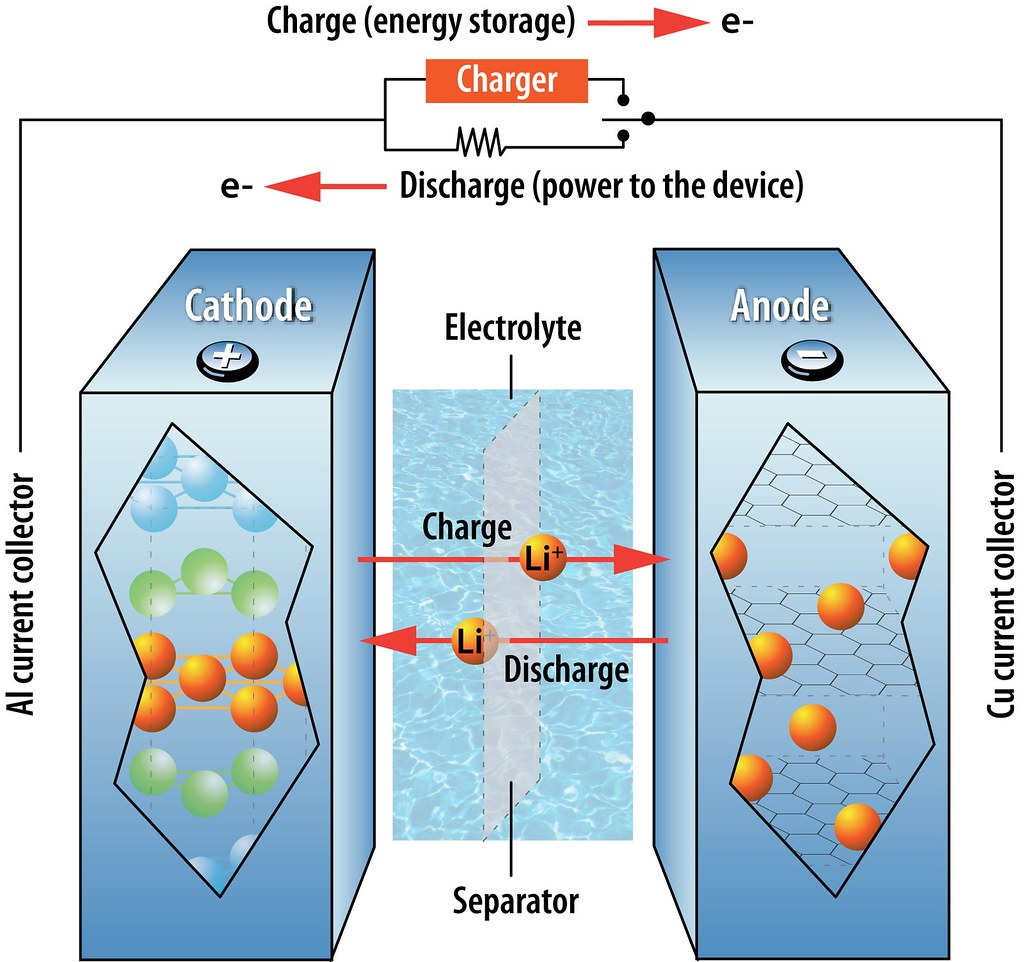
Separator
The separator is a thin, porous membrane that separates the anode and cathode of a lithium-ion battery. The separator allows the movement of lithium ions between the two electrodes while preventing the mixing of the anode and cathode materials. The separator is typically made of a polyolefin material, such as polyethylene or polypropylene.
The separator is an essential component in a lithium-ion battery that separates the anode and cathode while enabling the transport of lithium ions between them. The separator serves as a safety feature and helps prevent short circuits that can lead to battery failure or even fires. It must be mechanically strong, chemically stable, and have high ionic conductivity. In this article, we will discuss separator materials used in lithium-ion batteries.
Types of Separator Materials
- Polyolefin is a widely used separator material in lithium-ion batteries due to its low cost, high melting point, and good mechanical strength. It is made of a microporous membrane consisting of polyethylene and polypropylene. It has high chemical stability and good thermal stability, which makes it suitable for use in high-temperature environments.
- Ceramic separators are made of inorganic materials such as aluminum oxide, silicon dioxide, and lithium aluminum titanium phosphate (LATP). They have high thermal stability, good mechanical strength, and excellent chemical stability. Ceramic separators have a high melting point, which makes them suitable for use in high-temperature environments. They also have low electrical conductivity, which helps prevent short circuits.
- Polymer separators are made of polymers such as polyvinylidene fluoride (PVDF), polyethylene oxide (PEO), and polyacrylonitrile (PAN). They have high mechanical strength, good flexibility, and excellent chemical stability. They also have a high melting point, which makes them suitable for use in high-temperature environments. Polymer separators have a low electrical conductivity, which helps prevent short circuits.
- Composite separators are made of a combination of different separator materials. They are designed to combine the advantages of each material to provide better overall performance. For example, a composite separator may combine the high ionic conductivity of a polymer separator with the thermal stability of a ceramic separator.
Factors Affecting Separator Performance
- Pore Size: The pore size of a separator affects its ability to transport lithium ions. If the pores are too small, the lithium ions will not be able to pass through, and the battery’s performance will be affected. If the pores are too large, the separator may not provide adequate mechanical strength.
- Porosity: The porosity of a separator affects its ability to allow the flow of electrolytes. A separator with high porosity can allow a higher flow rate of electrolyte, which can improve the battery’s performance. However, if the porosity is too high, the mechanical strength of the separator may be compromised.
- Thickness: The thickness of a separator affects its ability to prevent short circuits. A thicker separator provides better insulation, but it can also increase the internal resistance of the battery, which can decrease its performance.
- Ionic conductivity: The ionic conductivity of a separator affects its ability to transport lithium ions. A separator with high ionic conductivity can improve the battery’s performance, but it can also increase the risk of short circuits.
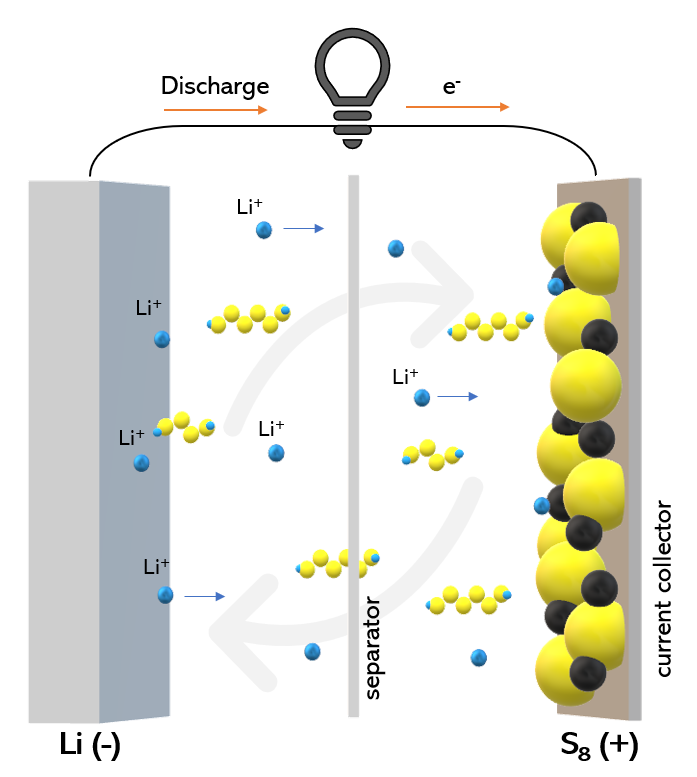
Conclusion
In conclusion, separator materials are an essential component of lithium-ion batteries. They help prevent short circuits, which can lead to battery failure or fires. The choice of separator material depends on the specific requirements of the battery, such as temperature range, mechanical strength, and ionic conductivity. Polyolefin, ceramic, polymer, and composite separators are the most commonly used separator materials. The pore size, porosity, thickness, and ionic conductivity of the separator affect its performance and must be carefully considered during the design of lithium-ion batteries.
Apart from these main components, lithium-ion batteries also require several other materials, such as conductive additives, binders, and current collectors, to ensure optimal performance and safety.
The production of these raw materials involves several processes, including mining, extraction, purification, and synthesis. For instance, the production of graphite anodes involves the mining of natural graphite or the synthesis of synthetic graphite from petroleum coke or coal tar pitch. Similarly, the production of metal oxide cathodes involves the extraction of raw materials such as lithium, cobalt, nickel, manganese, and iron, and their conversion into oxides.
The use of these raw materials in lithium-ion batteries has led to concerns about their sustainability and environmental impact. For instance, the mining and extraction of lithium and other metals can have negative impacts on local ecosystems and communities, and the disposal of spent batteries can lead to the release of toxic chemicals into the environment. As such, there is growing interest in developing more sustainable and environmentally friendly alternatives to lithium-ion batteries, such as solid-state batteries, sodium-ion batteries, and flow batteries.