Introduction
Electrochemical cells are devices that convert chemical energy into electrical energy or vice versa through redox reactions. They are essential components of batteries, fuel cells, and various other electrochemical systems. This article provides an overview of the basic principles underlying electrochemical cells, including their components, types, and operation.
An electrochemical cell is a mechanism that has the capability to produce electrical energy through the chemical reactions that transpire within it or employ the electrical energy that is furnished to it to expedite chemical reactions within it. These devices possess the ability to convert chemical energy to electrical energy, or conversely, electrical energy to chemical energy. An electrochemical cell that is frequently encountered is the standard 1.5-volt cell, which serves as a source of power for various electrical devices, including but not limited to television remote controls and clocks.
Cells that have the ability to produce electrical energy through chemical reactions are commonly referred to as Galvanic or Voltaic cells. Electrolytic cells are defined as cells in which chemical reactions are induced by the passage of an electric current.
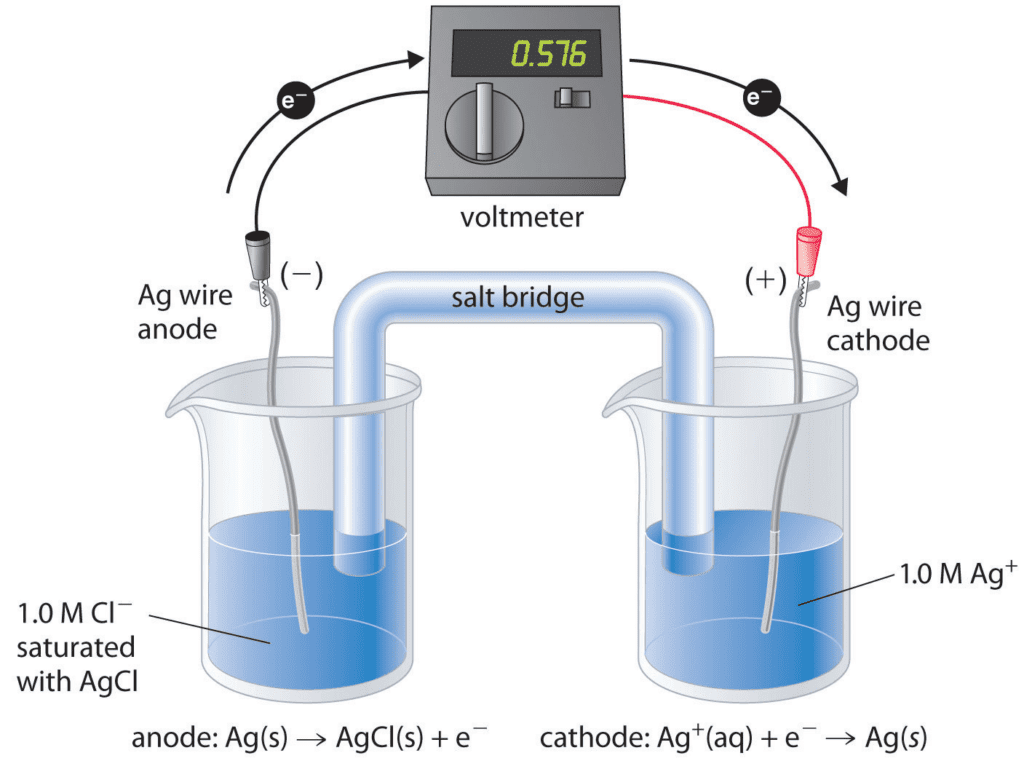
Components of Electrochemical Cells An electrochemical cell consists of two electrodes immersed in an electrolyte solution. The electrodes can be made of different materials, such as metals or conductive oxides, depending on the specific application. The electrolyte is typically a solution containing ions that facilitate the movement of charge between the electrodes.
The electrode at which oxidation occurs is called the anode, while the electrode at which reduction occurs is called the cathode. These processes involve the transfer of electrons from the anode to the cathode through an external circuit. The flow of electrons generates an electric current. In general, electrochemical cells consisted of two electrodes which are Anode and Cathode in a cell. The difference between Anode and Cathode are given below in table form.
Certainly! Here’s a table highlighting the key features of the cathode and the anode:
Features | Cathode | Anode |
---|---|---|
Location | Reduction | Oxidation |
Charge | Negative | Positive |
Electron Flow | Accepts electrons | Releases electrons |
Electrode Material | Material that gains electrons (electron moves in towards the cathode) | Material that loses electrons (electron moves out from Anode) |
Half-Reaction | Reduction half-reaction occurs | Oxidation half-reaction occurs |
Mass Change | Gains mass | Loses mass |
Significance | Site of the oxidation reaction | The copper electrode in a galvanic cell |
Example | Copper electrode in a galvanic cell | The Zinc electrode in a galvanic cell |
Note: It’s important to mention that the features may vary depending on the specific electrochemical system and the direction of electron flow. The table provides a general overview of the characteristics typically associated with the cathode and anode in a galvanic cell.
Types of Electrochemical Cells There are two main types of electrochemical cells
Galvanic (voltaic) cells and Electrolytic cells
Galvanic cells
These cells generate electrical energy from a spontaneous redox reaction. The anode is the site of oxidation and has a negative charge, while the cathode is the site of reduction and has a positive charge. The salt bridge or ion-permeable membrane connects the two half-cells and maintains charge neutrality. Common examples include alkaline batteries and lead-acid batteries.
Galvanic cells, also known as voltaic cells, are a type of electrochemical cell that converts chemical energy into electrical energy through spontaneous redox reactions. They are commonly found in batteries and have various practical applications. This article provides an overview of galvanic cells, including their components, working principles, and applications.
Components of Galvanic Cells: A galvanic cell consists of several key components:
- Anode: The anode is the electrode where oxidation takes place. It releases electrons into the external circuit. It is typically made of a metal or material that easily loses electrons.
- Cathode: The cathode is the electrode where reduction takes place. It attracts the electrons from the anode through the external circuit. It is usually made of a material that readily gains electrons.
- Electrolyte: The electrolyte is a solution that contains ions. It allows the movement of ions between the anode and cathode, completing the electrical circuit. The electrolyte can be an aqueous solution or a non-aqueous solution, depending on the specific cell design.
- Salt Bridge: The salt bridge or ion-permeable membrane connects the anode and cathode compartments. It maintains charge neutrality by allowing the flow of ions between the two compartments while preventing the mixing of solutions.
Working Principles of Galvanic Cells: The operation of a galvanic cell involves the following steps:
- Oxidation: At the anode, the electrode undergoes oxidation, releasing electrons into the external circuit. The metal atoms or ions at the anode lose electrons and form metal cations.
- Reduction: At the cathode, the electrode undergoes reduction, accepting electrons from the external circuit. The metal cations in the electrolyte gain electrons and deposit them as metal atoms or ions on the cathode.
- Electron Flow: Electrons flow from the anode to the cathode through the external circuit. This flow of electrons generates an electric current that can be used to power devices.
- Ion Flow: To maintain charge neutrality, ions from the electrolyte flow through the salt bridge or ion-permeable membrane to balance the charges between the anode and cathode compartments.
Cell Potential and Spontaneity: The cell potential, also known as the electromotive force (EMF) or voltage, is a measure of the electrochemical potential difference between the anode and cathode. It determines the ability of a galvanic cell to drive electrons through the external circuit. The cell potential is influenced by the nature of the electrode materials and the concentrations of the species involved.
In a galvanic cell, the redox reaction occurs spontaneously, meaning it releases energy. The cell potential can be determined by the difference in standard reduction potentials (E°) of the anode and cathode half-reactions. The greater the difference, the higher the cell potential, and the more energy the cell can produce.
Applications of Galvanic Cells
Galvanic cells have various practical applications, including:
- Batteries: Galvanic cells serve as the fundamental building blocks of batteries. They provide portable and rechargeable sources of electrical energy for devices ranging from small electronics to electric vehicles. Examples of galvanic cell-based batteries include alkaline batteries, lead-acid batteries, and lithium-ion batteries.
- Portable Power: Galvanic cells are commonly used in portable devices like smartphones, laptops, and cameras to provide a convenient and reliable power source.
- Emergency Power: Galvanic cells, such as zinc-air batteries, are used in emergency devices like backup power supplies, emergency lighting, and medical equipment.
- Environmental Monitoring: Galvanic cells find applications in environmental monitoring sensors, such as pH sensors and gas sensors.
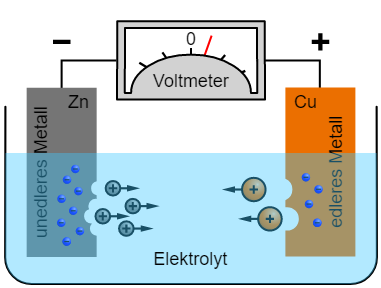
Electrolytic cells
In contrast to galvanic cells, electrolytic cells require an external source of electrical energy to drive a non-spontaneous redox reaction. The anode becomes the positive electrode, attracting negative ions for oxidation, while the cathode becomes the negative electrode, attracting positive ions for reduction. Electrolytic cells are used for processes such as electroplating and electrolysis. Electrolytic cells are electrochemical devices that use an external source of electrical energy to drive non-spontaneous redox reactions. They play a crucial role in various industrial processes, including electroplating, metal refining, and electrolysis. This article provides an in-depth overview of electrolytic cells, including their components, working principles, and applications.
Components of Electrolytic Cells
An electrolytic cell consists of the following essential components:
- Anode: The anode in an electrolytic cell is the positive electrode where oxidation takes place. It attracts negatively charged ions (anions) from the electrolyte and releases electrons into the external circuit.
- Cathode: The cathode in an electrolytic cell is the negative electrode where reduction takes place. It attracts positively charged ions (cations) from the electrolyte and accepts electrons from the external circuit.
- Electrolyte: The electrolyte in an electrolytic cell is a solution or molten compound that contains ions. It enables the conduction of electricity between the anode and cathode. The electrolyte can be an aqueous solution or a non-aqueous solution, depending on the specific application.
- External Power Source: Unlike galvanic cells, electrolytic cells require an external source of electrical energy, such as a battery or power supply, to drive the non-spontaneous reactions.
Working Principles of Electrolytic Cells
The operation of an electrolytic cell involves the following steps:
- Electrical Energy Supply: The external power source supplies electrical energy to the cell, creating a potential difference between the anode and cathode.
- Ion Migration: When the electrical current is applied, positive ions (cations) migrate toward the cathode, while negative ions (anions) migrate toward the anode through the electrolyte. This movement of ions helps maintain charge neutrality.
- Electrode Reactions: At the anode, oxidation occurs, resulting in the loss of electrons. The anode reaction is non-spontaneous and requires energy input from the external power source. At the cathode, reduction takes place, involving the gain of electrons. The cathode reaction is also non-spontaneous and driven by the external energy supply.
- Product Formation: The reactions at the anode and cathode lead to the formation of new substances or the modification of existing substances. These products are crucial for various industrial processes. For example, in electroplating, a metal coating is deposited on a substrate at the cathode.
Applications of Electrolytic Cells
Electrolytic cells find a wide range of applications in various industries:
- Electroplating: Electrolytic cells are extensively used in electroplating processes to deposit a layer of metal onto a surface. This technique is employed to enhance the appearance, durability, and corrosion resistance of objects, such as jewelry, automobile parts, and electronic components.
- Metal Refining: Electrolytic cells play a crucial role in metal refining processes. For example, electrolysis is used to purify copper by extracting it from impure copper ores. Similarly, electrolysis is utilized in the refining of other metals, including aluminum and zinc.
- Chlor-Alkali Industry: Electrolytic cells are vital in the production of chlorine gas, sodium hydroxide (caustic soda), and hydrogen gas, which are essential chemicals in various industries. The electrolysis of brine (sodium chloride solution) leads to the formation of these products.
- Water Electrolysis: Electrolytic cells are employed in the process of water electrolysis to generate hydrogen gas and oxygen
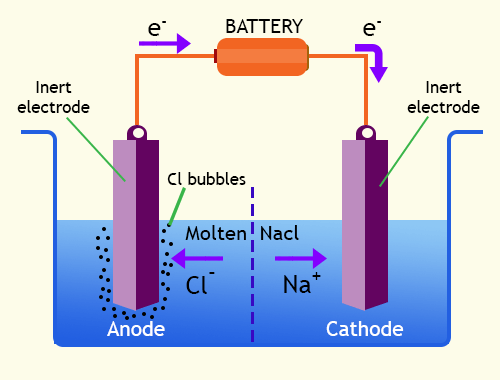
Cell Potential and Electrode Reactions
The cell potential, also known as the electromotive force (EMF) or voltage, is a measure of the electrochemical potential difference between the electrodes. It indicates the ability of a cell to drive electrons through an external circuit.
The electrode reactions in an electrochemical cell are governed by the thermodynamic principles of redox reactions. Each electrode reaction has a standard reduction potential, which is a measure of the tendency of a species to gain electrons. The overall cell potential is determined by the difference in reduction potentials between the anode and cathode reactions.
The Nernst equation relates the cell potential to the concentrations of the reactants and products involved in the electrode reactions. It allows the calculation of the cell potential under non-standard conditions, taking into account factors such as temperature and concentration gradients.
What are Primary and Secondary Cells?
Primary Cells
Primary cells, also known as primary batteries, are non-rechargeable electrochemical cells. They are designed to be used once and then discarded. Primary cells convert chemical energy directly into electrical energy through spontaneous redox reactions. The most common types of primary cells include alkaline batteries, zinc-carbon batteries, and lithium batteries. Primary cells are widely used in devices that have low power requirements and do not require frequent battery replacement.
Advantages of Primary Cells
- Convenience: Primary cells are ready to use and do not require charging. They can be easily replaced when they are depleted.
- Shelf Life: Primary cells have a long shelf life and can retain their charge for an extended period, even when not in use. This makes them suitable for applications where long-term storage is required.
- Reliability: Primary cells provide a consistent and reliable source of power. They deliver a steady voltage output until the chemical reactions are exhausted.
- Safety: Primary cells are generally considered safe to use, as they are less prone to leakage or overheating compared to some rechargeable cells.
Secondary Cells
Secondary cells, also known as secondary batteries or rechargeable batteries, are electrochemical cells that can be recharged and reused multiple times. They have the ability to reverse the chemical reactions that occur during discharge, allowing them to be charged again by supplying electrical energy. Common examples of secondary cells include lead-acid batteries, nickel-metal hydride (NiMH) batteries, and lithium-ion (Li-ion) batteries.
Advantages of Secondary Cells
- Rechargeability: The primary advantage of secondary cells is their ability to be recharged and reused multiple times. They can be connected to an external power source and restored to their original state, allowing for cost-effective and sustainable power solutions.
- Energy Density: Secondary cells generally have a higher energy density compared to primary cells, which means they can store more energy per unit weight or volume. This makes them suitable for applications that require higher power capacities.
- Environmental Impact: The reusability of secondary cells reduces the number of batteries that need to be disposed of, resulting in less environmental waste. They contribute to reducing the overall environmental impact associated with battery disposal.
- Cost-effectiveness: Although secondary cells may have a higher initial cost compared to primary cells, their ability to be recharged and reused makes them more cost-effective in the long run. They eliminate the need for frequent battery replacement.
Applications of Primary and Secondary Cells
Primary cells are commonly used in devices such as remote controls, flashlights, smoke detectors, and medical devices. They are ideal for applications with low power requirements and where the convenience of replacement outweighs the need for reusability.
Secondary cells find widespread use in applications such as portable electronics (e.g., smartphones, laptops, tablets), electric vehicles, renewable energy storage systems, and backup power supplies. Their ability to be recharged makes them suitable for applications where a reliable and reusable power source is needed.
Applications of Electrochemical Cells
Electrochemical cells have numerous practical applications:
- Batteries: Electrochemical cells are the basis of various battery systems, providing portable and rechargeable sources of electrical energy. Examples include lithium-ion batteries, nickel-metal hydride batteries, and fuel cells.
- Fuel Cells: Fuel cells generate electricity through the direct conversion of the chemical energy of a fuel, such as hydrogen or methanol. They offer high energy efficiency and low environmental impact, making them promising alternatives for transportation and stationary power generation.
- Corrosion Protection: Electrochemical cells can be utilized to protect metal structures from corrosion. By employing sacrificial anodes made of more easily oxidizable metals, the anode material corrodes instead of the protected metal.
- Sensors and Electrolysis: Electrochemical cells find applications in various sensing technologies, such as pH sensors and gas sensors. They are also employed in electrolysis processes, which involve the decomposition of compounds using an electric current, such as a water-electrolyte.