Electrolysis is a green revolution
Introduction
Electrolysis can be considered a green revolution because it enables the production of hydrogen, a clean and renewable energy carrier, by using renewable electricity to split water into its constituent elements, hydrogen, and oxygen. This process is known as water electrolysis and is considered to be a sustainable method for producing hydrogen, as it produces no greenhouse gases or other harmful emissions.
Hydrogen produced through electrolysis can be used as a fuel in fuel cells to generate electricity, or as a feedstock for the production of chemicals and other materials. When used in fuel cells, hydrogen can produce electricity with high efficiency and zero emissions, making it an attractive option for powering a range of applications, from cars and trucks to buildings and factories.
In addition to producing green hydrogen, electrolysis can also be used for the production of other valuable chemicals and materials, such as chlorine and sodium hydroxide, which are used in a wide range of industries, including chemical manufacturing, water treatment, and paper production.
Overall, the use of electrolysis for the production of green hydrogen and other materials has the potential to contribute significantly to the transition to a low-carbon, sustainable economy.
The Hydrogen Energy Earth shot target of decreasing the cost of clean hydrogen by 80% to $1 per kilogram in a decade can be achieved by electrolysis (“1 1 1”). No greenhouse gas emissions are possible if the power utilized is generated from renewable sources. It is important to take into account the cost and efficiency of the power needed to produce hydrogen by electrolysis, as well as the emissions that are generated as a consequence of the creation of electricity. There are many places in the nation where today’s power infrastructure does not meet the needs for electrolysis because of the quantity of fuel needed for electricity production and the greenhouse gas emissions that result from this process. Renewable (wind, solar, hydro, geothermal) and nuclear energy sources are pursuing hydrogen generation through electrolysis. A large reduction in the cost of hydrogen generation is needed in order to compete with carbon-based methods like natural gas reforming, which produce essentially negligible greenhouse gas and criterion pollutant emissions.
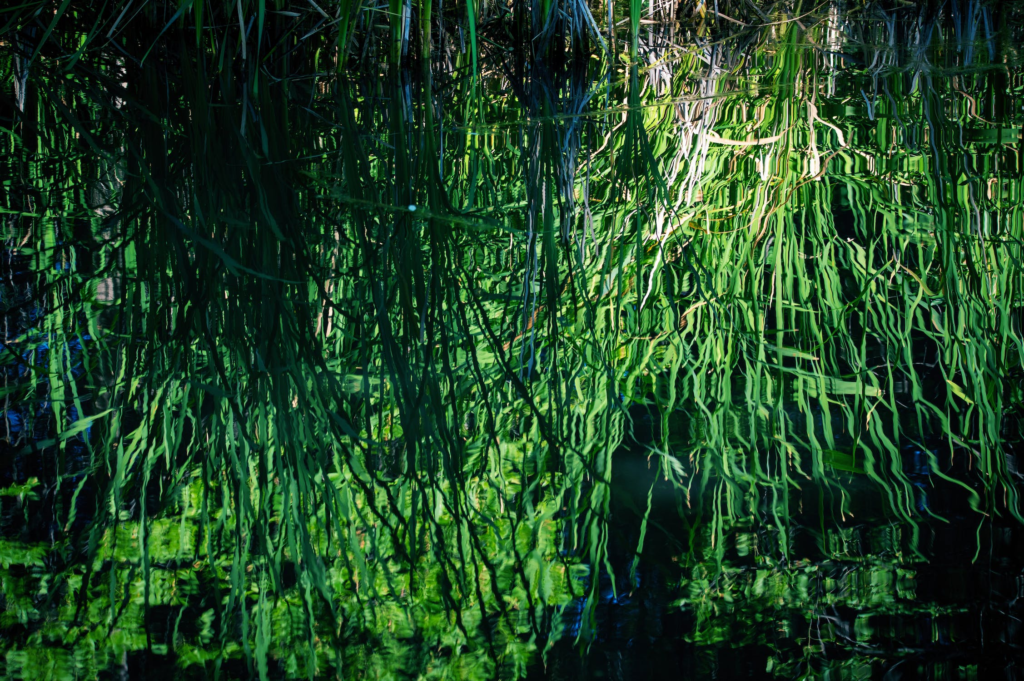
Different Resources of Hydrogen Production
Electrolysis is a process that involves the use of electricity to split water molecules into hydrogen and oxygen. This hydrogen can then be used as a clean, renewable energy source for fuel cells or other applications. Here are some reasons why electrolysis is a good option for the green revolution:
The renewable energy source from Hydrogen
Electrolysis uses electricity, which can be generated from renewable energy sources such as wind, solar, or hydroelectric power. This means that the hydrogen produced by electrolysis can be considered a renewable energy source, as it is generated using clean, sustainable electricity.
Hydrogen is a versatile energy carrier that can be produced from a variety of sources, including renewable energy sources such as wind, solar, and hydropower. When produced from renewable energy sources, hydrogen is known as green hydrogen, as it is a clean and sustainable energy carrier that produces no greenhouse gas emissions.
One of the most common ways to produce green hydrogen is through a process called water electrolysis. Water electrolysis involves using an electric current to split water molecules into their constituent elements, hydrogen, and oxygen. The electricity used for water electrolysis can be generated from renewable sources, such as wind or solar power, to produce green hydrogen.
There are two main types of water electrolysis: alkaline electrolysis and proton exchange membrane (PEM) electrolysis. Alkaline electrolysis is the more established and widely used technology, while PEM electrolysis is a newer technology that is gaining popularity due to its high efficiency and flexibility.
Alkaline electrolysis involves using an alkaline solution, typically potassium hydroxide (KOH) or sodium hydroxide (NaOH), as the electrolyte. The electrolysis cell contains an anode and a cathode, which are separated by a membrane. When an electric current is passed through the cell, water molecules are split into hydrogen ions and hydroxide ions. The hydrogen ions migrate to the cathode, where they combine with electrons from the electric current to form hydrogen gas, while the hydroxide ions combine with the electrons at the anode to form oxygen gas.
PEM electrolysis uses a polymer membrane as the electrolyte instead of an alkaline solution. The membrane allows the hydrogen ions to pass through while preventing the passage of oxygen gas. This results in higher efficiency and lower energy consumption compared to alkaline electrolysis. PEM electrolysis also has the advantage of being able to operate at lower temperatures and pressures, which makes it more flexible and easier to integrate into a range of applications.
Green hydrogen can also be produced from renewable sources such as biomass and biogas through a process called steam reforming or gasification. Biomass and biogas are first converted into a mixture of hydrogen and carbon monoxide, known as syngas, which is then separated into pure hydrogen and carbon dioxide. This process can be carbon-neutral if the carbon dioxide produced is captured and stored, resulting in a net-zero emission of greenhouse gases.
Once produced, green hydrogen can be stored and transported using a range of methods, including compressed gas, liquefaction, or chemical storage. It can also be used as a fuel in a range of applications, including fuel cell vehicles, power generation, and industrial processes.
Fuel cells are electrochemical devices that convert the chemical energy of hydrogen into electricity. When hydrogen is supplied to the anode of a fuel cell, it reacts with oxygen from the air supplied to the cathode to produce water and electricity. Fuel cells have high efficiency, low emissions, and can operate at a range of scales, making them a promising technology for a range of applications, from powering vehicles to providing backup power for buildings and homes.
In addition to fuel cells, green hydrogen can also be used as a feedstock for the production of chemicals and materials, such as ammonia, methanol, and plastics. The production of these chemicals from green hydrogen can provide a sustainable and low-carbon alternative to traditional petrochemical-based processes.
In conclusion, the production of green hydrogen from renewable energy sources offers a promising pathway toward a low-carbon and sustainable energy future. With the growing deployment of renewable energy sources such as wind and solar power, the production of green hydrogen is becoming increasingly feasible and cost-effective, offering a clean and versatile energy carrier that can help reduce greenhouse gas emissions and address the challenges of climate change.
Carbon-free energy from Hydrogen
When hydrogen is burned, it produces only water and no harmful emissions or greenhouse gases. This makes hydrogen a carbon-free energy source that can be used to power vehicles or generate electricity without contributing to climate change.
Hydrogen has the potential to play a significant role in the transition to a carbon-free energy system. Hydrogen can be produced using renewable energy sources and can be used as a fuel in a variety of applications, including transportation, power generation, and industrial processes. When hydrogen is used as a fuel, it produces no greenhouse gas emissions, making it an attractive option for reducing carbon emissions.
One of the most common ways to produce hydrogen is through a process called water electrolysis. Water electrolysis involves using an electric current to split water molecules into their constituent elements, hydrogen and oxygen. The electricity used for water electrolysis can be generated from renewable sources, such as wind or solar power, to produce carbon-free hydrogen.
Once produced, carbon-free hydrogen can be stored and transported using a range of methods, including compressed gas, liquefaction, or chemical storage. It can also be used as a fuel in a range of applications, including fuel cell vehicles, power generation, and industrial processes.
Fuel cells are electrochemical devices that convert the chemical energy of hydrogen into electricity. When hydrogen is supplied to the anode of a fuel cell, it reacts with oxygen from the air supplied to the cathode to produce water and electricity. Fuel cells have high efficiency, low emissions, and can operate at a range of scales, making them a promising technology for a range of applications, from powering vehicles to providing backup power for buildings and homes.
In addition to fuel cells, carbon-free hydrogen can also be used as a feedstock for the production of chemicals and materials, such as ammonia, methanol, and plastics. The production of these chemicals from carbon-free hydrogen can provide a sustainable and low-carbon alternative to traditional petrochemical-based processes.
One challenge with the production of hydrogen is that it can be energy-intensive, particularly if the hydrogen is produced using fossil fuels. However, the use of renewable energy sources to produce hydrogen can reduce the carbon footprint of the production process. In addition, new technologies are being developed to increase the efficiency and reduce the cost of hydrogen production, making it more competitive with traditional fossil fuels.
Another challenge with hydrogen is its storage and transportation. Hydrogen has a low energy density, which means that it takes up more space than traditional fuels. This can make it challenging to store and transport large quantities of hydrogen. However, new storage and transportation technologies are being developed, such as hydrogen fuel cells and pipelines, that can help overcome these challenges.
In summary, the production and use of carbon-free hydrogen offers a promising pathway towards a carbon-free energy future. With the growing deployment of renewable energy sources such as wind and solar power, the production of carbon-free hydrogen is becoming increasingly feasible and cost-effective, offering a clean and versatile energy carrier that can help reduce greenhouse gas emissions and address the challenges of climate change. While there are still challenges to overcome, the potential benefits of carbon-free hydrogen make it an important technology to watch in the coming years.
Energy storage from Hydrogen
Energy storage is a crucial aspect of transitioning to a renewable energy system, as renewable energy sources such as wind and solar power are intermittent and can fluctuate based on weather conditions. Hydrogen can be used as an energy storage solution in a number of different applications, including power generation, transportation, and industrial processes.
One of the most common ways to store hydrogen is in a compressed gas form. Hydrogen can be compressed and stored in tanks, much like compressed natural gas (CNG) or propane. Compressed hydrogen can then be used as a fuel for vehicles, or as a source of energy for power generation or industrial processes. However, compressed hydrogen has a low energy density and requires high-pressure storage, making it less efficient for some applications.
Another method for storing hydrogen is through liquefaction. Hydrogen can be cooled to a temperature of -253°C, at which point it becomes a liquid with a much higher energy density than compressed gas. Liquid hydrogen can be stored in tanks and transported over long distances. However, the process of liquefying hydrogen is energy-intensive and can be expensive, making it less practical for some applications.
A promising method for storing hydrogen is through chemical storage. Hydrogen can be combined with other elements to form molecules such as ammonia or methanol, which can be stored and transported more easily than hydrogen gas or liquid. These molecules can then be converted back into hydrogen when needed, providing a flexible and efficient method for hydrogen storage.
In addition to these storage methods, hydrogen can also be used as a storage medium for renewable energy sources. Excess renewable energy can be used to produce hydrogen through water electrolysis, which can then be stored for later use. This stored hydrogen can then be used to generate electricity through fuel cells or combustion engines, providing a source of energy that is carbon-free and can help balance the intermittent nature of renewable energy sources.
Hydrogen storage is also an important consideration for transportation applications. Fuel cell vehicles use hydrogen as a fuel, which is stored in high-pressure tanks onboard the vehicle. As fuel cell vehicles become more widespread, the development of efficient and cost-effective hydrogen storage solutions will be crucial to their success.
One of the key challenges with hydrogen storage is safety. Hydrogen is a highly flammable gas and requires special safety measures to ensure its safe storage and handling. This includes specialized storage tanks, safety valves, and monitoring systems to detect leaks or other safety hazards.
Another challenge is the cost of hydrogen storage. While hydrogen storage technologies have improved in recent years, they are still more expensive than traditional energy storage solutions, such as batteries or pumped hydro storage. However, the cost of hydrogen storage is expected to decrease as the technology advances and becomes more widespread.
In conclusion, hydrogen offers a promising solution for energy storage in a renewable energy system. With a range of storage options, including compressed gas, liquefaction, chemical storage, and as a storage medium for excess renewable energy, hydrogen can provide a flexible and efficient source of energy that can help balance the intermittent nature of renewable energy sources. While there are still challenges to overcome, such as safety and cost, the potential benefits of hydrogen storage make it an important technology to watch in the coming years.
Versatility from Hydrogen
Hydrogen is one of the most versatile elements on earth and has the potential to be a key player in a wide range of applications. Its versatility stems from its unique properties, including its high energy content, low environmental impact, and ability to be stored and transported in a variety of forms. In this article, we will explore some of the key applications and benefits of hydrogen’s versatility.
- Transportation
One of the most promising applications of hydrogen is in the transportation sector. Fuel cell vehicles powered by hydrogen are becoming increasingly popular, with major car manufacturers investing in the development of hydrogen fuel cell technology. These vehicles emit only water vapor and have a longer driving range than battery electric vehicles. Additionally, hydrogen can be used as a fuel for other transportation modes, such as buses, trains, and ships.
- Energy Storage
As mentioned in a previous article, hydrogen can be used as an energy storage solution, particularly for renewable energy sources. The excess renewable energy can be used to produce hydrogen through water electrolysis, which can then be stored and used to generate electricity through fuel cells or combustion engines.
- Heating and Cooling
Hydrogen can be used as a fuel for heating and cooling applications. For example, hydrogen can be used in fuel cells to generate electricity and heat for residential and commercial buildings. It can also be used as a feedstock for the production of ammonia, which can be used as a refrigerant in air conditioning systems.
- Industrial Processes
Hydrogen can be used as a feedstock for a wide range of industrial processes, including the production of ammonia, methanol, and other chemicals. It can also be used in the steel and glass industries as a reducing agent to remove oxygen and impurities from raw materials.
- Power Generation
Hydrogen can be used to generate electricity through fuel cells or combustion engines. This can provide a clean and efficient source of power for a range of applications, including backup power for critical infrastructure, distributed power generation, and remote power systems.
- Agriculture
Hydrogen can be used in agriculture as a fertilizer, reducing the need for synthetic fertilizers that contribute to greenhouse gas emissions. Hydrogen can also be used to power agricultural equipment, reducing emissions and increasing efficiency.
- Space Exploration
Hydrogen has been used as a fuel for space exploration for decades. The Space Shuttle and other spacecraft have used hydrogen fuel cells to generate electricity and water for astronauts on board. Additionally, hydrogen can be used as a propellant for rockets, providing a clean and efficient alternative to traditional rocket fuels.
- Decarbonization
Finally, hydrogen has the potential to play a key role in decarbonizing a range of sectors, including transportation, industry, and power generation. By using hydrogen as a fuel or feedstock, emissions can be greatly reduced, contributing to global efforts to combat climate change.
In conclusion, the versatility of hydrogen makes it a valuable resource for a wide range of applications. From transportation to industrial processes, power generation to space exploration, hydrogen has the potential to provide clean and efficient solutions to some of the world’s most pressing challenges. While there are still challenges to overcome, such as safety, cost, and infrastructure development, the versatility of hydrogen makes it an important technology to watch in the coming years.
Electrolysis Efficiency from Hydrogen
Electrolysis is the process of using electricity to split water molecules into hydrogen and oxygen. The efficiency of this process is an important factor in determining the viability of using hydrogen as a clean energy source. In this article, we will explore the factors that affect the efficiency of electrolysis and the current state of the technology.
The efficiency of electrolysis is measured by the amount of energy required to produce a given amount of hydrogen. This is known as the “energy efficiency” of the process. The energy efficiency is influenced by several factors, including the type of electrolyzer, the operating conditions, and the source of electricity used.
- Type of Electrolyzer
There are several types of electrolyzers used for hydrogen production, including alkaline electrolyzers, polymer electrolyte membrane (PEM) electrolyzers, and solid oxide electrolyzers. Each type has different efficiency characteristics.
Alkaline electrolyzers are the most mature and widely used technology. They typically have an energy efficiency of around 70-80%, but can be as high as 90% in some cases. Alkaline electrolyzers are relatively inexpensive and can handle a wide range of operating conditions.
PEM electrolyzers are newer technology and are becoming increasingly popular due to their high efficiency and scalability. They typically have an energy efficiency of around 80-90%, but can be as high as 95% in some cases. PEM electrolyzers require high-purity water and operate at high pressures, which can increase costs.
Solid oxide electrolyzers are another emerging technology that has the potential for high efficiency. They typically have an energy efficiency of around 80-90%, but can be as high as 95% in some cases. Solid oxide electrolyzers operate at high temperatures, which can increase costs, but can also provide additional benefits such as the ability to use waste heat for steam generation.
- Operating Conditions
The operating conditions of an electrolyzer can also affect its efficiency. The temperature, pressure, and electrolyte concentration can all impact the energy efficiency of the process.
Increasing the temperature of the electrolysis process can improve efficiency by reducing the energy required to split water molecules. However, higher temperatures can also increase the risk of corrosion and other issues.
Increasing the pressure of the electrolysis process can also improve efficiency by increasing the concentration of hydrogen and oxygen in the gas stream. However, higher pressures can also increase the risk of leaks and other safety issues.
Optimizing the concentration of the electrolyte can also improve efficiency by reducing the resistance of the electrolyte to the flow of electricity. However, too high of a concentration can lead to corrosion and other issues.
- Source of Electricity
The source of electricity used for electrolysis can also affect the efficiency of the process. The most common sources of electricity used for electrolysis are grid electricity and renewable electricity.
Grid electricity is typically the cheapest and most widely available source of electricity. However, it is also typically the least clean, as it is often generated from fossil fuels.
Renewable electricity, such as solar or wind power, is a cleaner source of electricity that can greatly reduce the carbon footprint of hydrogen production. However, the availability and cost of renewable electricity can vary depending on location and time of day.
The efficiency of electrolysis is a critical factor in determining the viability of using hydrogen as a clean energy source. While current technologies can achieve energy efficiencies of around 70-90%, there is still room for improvement. Emerging technologies such as solid oxide electrolyzers and advances in operating conditions and renewable energy sources hold promise for increasing the efficiency of electrolysis in the future. As the demand for clean energy continues to grow, improving the efficiency of electrolysis will be an important area of focus for researchers and industry stakeholders.
Overall, electrolysis is a promising technology that has the potential to play a significant role in the green revolution. By producing clean, renewable hydrogen from renewable energy sources, electrolysis can help to reduce our dependence on fossil fuels and promote a more sustainable, low-carbon energy future.
Integration with renewable energy power production is a possibility:
The creation of hydrogen by electrolysis may provide chances for synergy with dynamic and intermittent power generation, both of which are characteristics of certain types of renewable energy technology. For instance, even if the cost of using wind power has been steadily going down, the inherent unpredictability of wind conditions remains a barrier to the efficient use of wind power. It is possible for a wind farm to combine the production of hydrogen fuel and electric power, which would allow for more flexibility in shifting output to most effectively match resource availability with system operating demands and market variables. In addition, when wind farms produce an excessive amount of electricity, rather than reducing the amount of electricity being produced as is customarily done, it is possible to use this excess electricity to produce hydrogen through the process of electrolysis. This is an alternative to the more common practice of reducing the amount of electricity being produced.
- Because the majority of the nation’s power comes from sources that are both significant generators of greenhouse gas emissions and significant consumers of energy, the electricity that comes from the grid in today’s world is not the best choice for use in electrolysis. The creation of electricity via the use of renewable or nuclear energy technologies, either independently from the grid or as a rising component of the grid mix, is one alternative that might be pursued in order to circumvent these constraints on the production of hydrogen by electrolysis.
- The United States Department of Energy and other organizations are continuing their work to reduce the cost of producing power from renewable sources and to improve the efficiency of producing electricity from fossil fuels by using carbon capture, utilization, and storage technologies. For instance, the generation of power from wind is expanding at a fast rate around the world, including in the United States.
Overcoming Obstacles Is the Purpose of Research
- Achieving the Hydrogen Shot clean hydrogen cost objective of $1/kg H2 by 2030 (and an intermediate target of $2/kg H2 by 2025) through a better understanding of the performance, cost, and durability trade-offs of electrolyzer systems under expected future dynamic operating modes utilizing CO2-free power.
- Making the electrolyzer unit and other system components more cost-effective.
- Achieving higher electricity-to-hydrogen conversion efficiency under a variety of operational situations.
- Development of mitigating measures to enhance the operating life of electrolyzer cells, stacks, and systems.
Half Cell Reactions:
There are two electrodes in a galvanic cell or a basic battery, and one of those electrodes is called a half cell. Zn-Cu batteries, for example, have two half-cells that form an oxidizing-reducing pair. A half cell is formed by dissolving a little amount of reactant in an electrolyte solution. An electric conductor and salt bridge must be used to link two half-cells in order for any reaction to occur.
On the Cathode, reduction takes place.
- Oxidant+ne−→ReductantOxidant+ne−→Reductant
- Example: Cu2++2e−→Cu and Cu2++2e−→Cu
- Cu2+\Cu2+ is the oxidizing agent and Cu Cu the reducing agent.
On the Anode, oxidation takes place.
- Reductant→Oxidant+ne−Reductant→Oxidant+ne−
- Example: Zn→Zn2++2e−, Zn→Zn2++2e−.
- Zn Zn is the reducing agent, and Zn2+ Zn2+ the oxidizing agent.
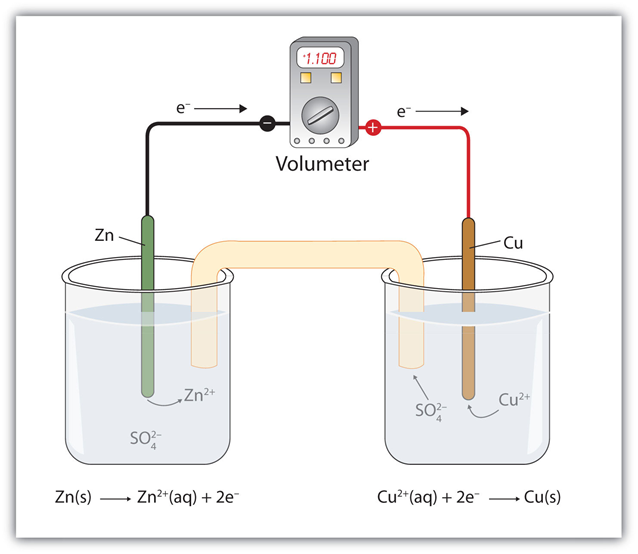
A battery cannot function without at least two electrodes: the anode, which is where oxidation takes place, and the cathode, which is where reduction takes place. Both oxidation and reduction are always necessary for the functioning of a battery.
An anode, a cathode, a load, and a salt bridge are necessary components for the functioning of a battery (if the salt bridge is not there already). These are the primary components that make up a battery.