Supercapacitor
Introduction
A supercapacitor, also known as an ultracapacitor, is an energy storage device that combines the characteristics of both capacitors and batteries. Unlike batteries, which store energy through a chemical reaction, supercapacitors store energy in an electric field created by the accumulation of charge on its plates.
Supercapacitors, also known as ultracapacitors or electrochemical capacitors, are a type of energy storage device that has gained significant attention in recent years due to their high power density, fast charging times, and long cycle life. Supercapacitors are capable of storing and delivering electrical energy quickly and efficiently, making them ideal for applications in which high power is required for short periods of time.
Supercapacitors are similar to traditional capacitors in that they both store energy in an electric field, but they differ in the way that they store that energy. Traditional capacitors store energy in the form of an electric field between two conducting plates separated by a dielectric material, while supercapacitors store energy in the form of an electrical double layer formed at the interface between a high-surface-area electrode and an electrolyte solution.
The high-surface-area electrode in a supercapacitor is typically made of activated carbon, which has a very porous structure that provides a large surface area for the electrical double layer to form. The electrolyte solution in a supercapacitor is typically an aqueous or organic solvent containing ions that can migrate to the electrode surface and form the electrical double layer.
Supercapacitors are designed to operate at low voltages, typically less than 3 volts, and have a capacitance ranging from a few Farads to several thousand Farads. The capacitance of a supercapacitor is determined by the surface area of the electrode, the distance between the electrode and the electrolyte, and the dielectric constant of the electrolyte.
One of the key advantages of supercapacitors is their high power density, which is the amount of power that can be delivered per unit of mass or volume. Supercapacitors have a much higher power density than batteries and can deliver bursts of power that are much higher than their rated capacity. This makes them ideal for applications in which high power is required for short periods of time, such as hybrid electric vehicles, electric bicycles, and power tools.
Supercapacitors also have a very fast charging time, typically a few seconds to a few minutes, which is much faster than batteries. This makes them ideal for applications in which rapid charging is required, such as regenerative braking systems in electric vehicles.
Another advantage of supercapacitors is their long cycle life, which is the number of charge-discharge cycles that they can withstand before their performance degrades. Supercapacitors have a much longer cycle life than batteries and can withstand tens of thousands of cycles without significant degradation in performance.
Supercapacitors have several disadvantages as well. They have a lower energy density than batteries, which means they cannot store as much energy per unit of mass or volume. This limits their usefulness in applications where energy storage is the primary requirement, such as grid-scale energy storage.
Supercapacitors also have a higher self-discharge rate than batteries, which means that they lose their charge more quickly when not in use. This limits their usefulness in applications where long-term energy storage is required.
In recent years, significant research efforts have been focused on developing new materials and designs for supercapacitors to overcome these limitations and improve their performance. Some of the areas of research include the development of new electrode materials, such as graphene and carbon nanotubes, the use of new electrolyte materials, such as ionic liquids, and the development of new device architectures, such as asymmetric and hybrid supercapacitors.
In conclusion, supercapacitors are important energy storage devices that offer several advantages over traditional batteries, including high power density, fast charging times, and long cycle life. While they have some limitations, significant research efforts are underway to overcome these limitations and improve their performance.
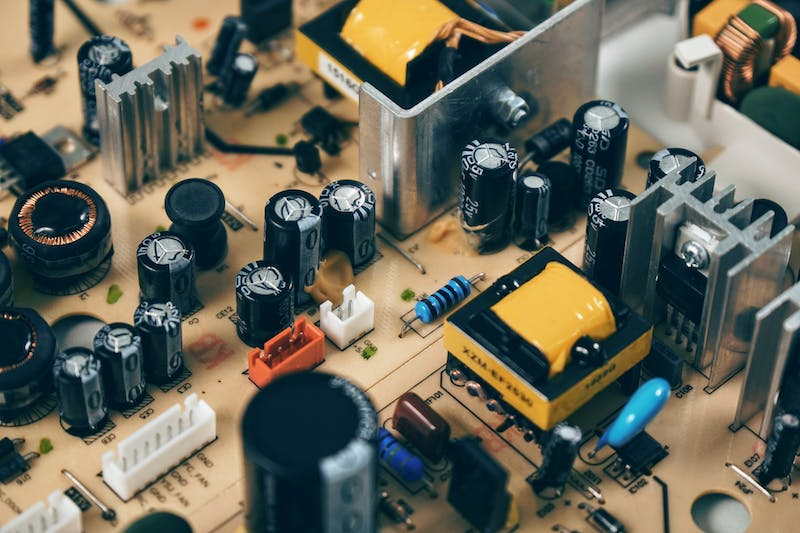
The basic structure of a supercapacitor consists of two electrodes separated by an electrolyte. The electrodes are made of a porous material with a high surface area, such as activated carbon, and are coated with a thin layer of conducting material, such as carbon nanotubes. The electrolyte is a solution of an organic or inorganic compound that can conduct ions.
When a voltage is applied to a supercapacitor, ions from the electrolyte accumulate at the surface of the electrodes, forming a double layer of charge. This process is called electrostatic adsorption, and it allows the supercapacitor to store electrical energy. The amount of energy that a supercapacitor can store is proportional to the surface area of the electrodes and the amount of charge that can be stored at the double layer.
The energy stored in a supercapacitor can be calculated using the equation: Energy = 1/2 * CV^2, where C is the capacitance and V is the voltage. Capacitance is a measure of the amount of charge that can be stored on the electrodes and is determined by the surface area of the electrodes, the separation between them, and the dielectric constant of the electrolyte.
Different types of Supercapacitors
Supercapacitors, also known as ultracapacitors or electrochemical capacitors, are energy storage devices that bridge the gap between conventional capacitors and batteries. Unlike traditional capacitors, supercapacitors store energy electrostatically instead of chemically. This allows them to charge and discharge much faster than batteries while also providing much higher power density. Supercapacitors have found numerous applications in the areas of transportation, renewable energy systems, and electronics.
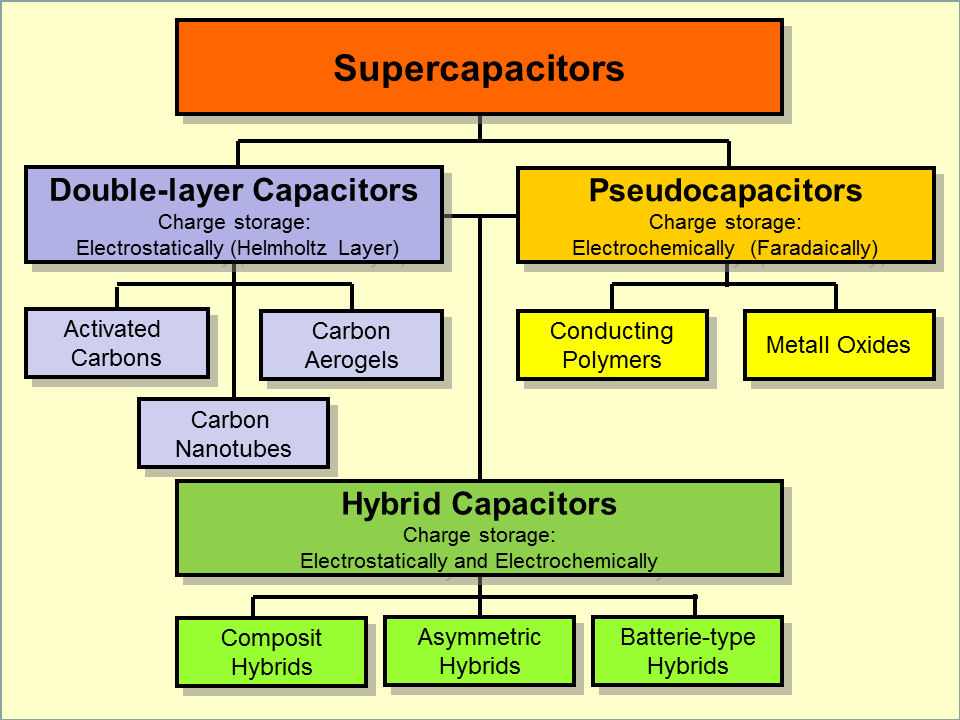
There are three main types of supercapacitors:
- Electrostatic Double-Layer Capacitors (EDLCs)
Electrostatic Double-Layer Capacitors (EDLCs), also known as electric double-layer capacitors or supercapacitors, are a type of energy storage device that operates based on the principle of electrostatic charge separation. EDLCs have gained significant attention in recent years due to their high power density, fast charging and discharging capabilities, and long cycle life. They can store much higher amounts of energy compared to conventional capacitors and can deliver the energy quickly when needed.
The EDLC structure consists of two electrodes separated by an electrolyte, which serves as a medium for the flow of ions. The electrodes are typically made of activated carbon, which has a high surface area and high porosity. The high surface area allows for a large amount of charge to be stored on the surface of the electrode, while the high porosity provides a large number of sites for ion adsorption.
The charge storage mechanism in EDLCs is based on the formation of an electric double layer at the electrode-electrolyte interface. When a voltage is applied across the electrodes, ions from the electrolyte are attracted to the surface of the electrodes and form a layer of charge. The electric double layer that is formed has a thickness of a few nanometers and can store a large amount of charge. The charge is stored electrostatically, meaning that no chemical reaction takes place during the charging or discharging process.
There are two types of EDLCs based on the type of electrolyte used: aqueous and non-aqueous. Aqueous EDLCs use water-based electrolytes, such as sulfuric acid, potassium hydroxide, or sodium chloride. These electrolytes have a high conductivity and are inexpensive, but they have a limited voltage range and low energy density. Non-aqueous EDLCs, on the other hand, use organic or inorganic solvents as electrolytes, such as acetonitrile or propylene carbonate. These solvents have a low viscosity and a high dielectric constant, which allows for a higher voltage range and energy density compared to aqueous electrolytes.
Overall, EDLCs have found applications in a wide range of fields, including electronics, transportation, and energy storage systems. They are particularly suitable for applications that require high power delivery and frequent charge and discharge cycles, such as hybrid electric vehicles and renewable energy systems. Ongoing research is focused on improving the energy density and stability of EDLCs to make them more competitive with other energy storage technologies.
- Pseudocapacitors
Pseudocapacitors are a type of electrochemical capacitor that use a combination of electrostatic and faradaic charge storage mechanisms to store electrical energy. They are also sometimes called redox supercapacitors because they rely on reversible redox reactions at the electrode/electrolyte interface to store energy.
The basic principle behind a pseudocapacitor is similar to that of an EDLC (Electrostatic Double-Layer Capacitor), which is the most common type of electrochemical capacitor. EDLCs store energy by accumulating charges at the interface between the electrode and the electrolyte, resulting in a double-layer of charge separation. Pseudocapacitors, on the other hand, use redox reactions at the electrode surface to store and release charge.
Pseudocapacitors typically use metal oxides or conducting polymers as the active electrode material. These materials have high surface areas and can undergo reversible redox reactions, which makes them ideal for use in energy storage devices. When the pseudocapacitor is charged, a redox reaction occurs at the surface of the electrode, resulting in the storage of charge. When the capacitor is discharged, the redox reaction is reversed, and the stored charge is released.
One of the main advantages of pseudocapacitors is that they have a higher energy density compared to EDLCs, which means they can store more energy per unit volume or mass. This is because the redox reactions that occur at the electrode surface allow for a greater amount of charge to be stored. Pseudocapacitors can also have a longer cycle life and be more stable over time compared to other types of electrochemical capacitors.
However, pseudocapacitors also have some limitations. They typically have lower power densities and longer charge/discharge times compared to EDLCs. This is because the redox reactions that occur during charging and discharging are slower compared to the electrostatic processes that occur in EDLCs. Pseudocapacitors are also more susceptible to degradation over time due to the redox reactions that occur at the electrode surface during cycling.
Despite these limitations, pseudocapacitors have a wide range of applications in energy storage systems. They are used in hybrid electric vehicles, where they can provide high-power bursts for acceleration, regenerative braking, and energy recovery. They are also used in renewable energy systems, where they can be used to store energy from solar panels or wind turbines for later use. Pseudocapacitors are also finding new applications in portable electronic devices, such as smartphones and laptops, where they can provide high-power bursts for rapid charging and data transfer.
In conclusion, pseudocapacitors are a promising technology for energy storage that combine the high energy density of batteries with the high power density of capacitors. They have the potential to revolutionize energy storage systems, making them more efficient, reliable, and cost-effective. However, further research is needed to optimize their performance and overcome the limitations associated with their use.
- Hybrid Supercapacitors
Hybrid supercapacitors are a class of energy storage devices that combine the high power density of electrostatic capacitors with the high energy density of batteries. They are designed to provide high power and energy performance in a single device, making them ideal for use in applications that require both high power and energy, such as electric vehicles, renewable energy systems, and portable electronics.
Hybrid supercapacitors consist of two main components: an electrochemical capacitor electrode and a battery electrode. The electrochemical capacitor electrode is usually made of activated carbon or other porous materials that can store a large amount of charge through the adsorption of ions on their surface. The battery electrode, on the other hand, is made of materials that undergo reversible redox reactions, such as metal oxides, conductive polymers, or transition metal dichalcogenides.
The working principle of hybrid supercapacitors is based on the combination of two charge storage mechanisms: the electrostatic double-layer capacitance and the pseudocapacitance. When a voltage is applied to the device, ions from the electrolyte are attracted to the surface of the electrochemical capacitor electrode, where they form an electrical double layer. This process creates a high capacitance, which allows the device to store a large amount of charge in a short period of time.
In addition, the battery electrode undergoes redox reactions during charge and discharge cycles, which leads to the storage of additional charge through the formation of pseudocapacitive species. These reactions allow the device to store energy at a much higher density than traditional electrostatic capacitors, while maintaining high power performance.
There are several types of hybrid supercapacitors, including asymmetric and symmetric devices. Asymmetric hybrid supercapacitors consist of two different electrodes with different electrochemical properties, such as an activated carbon electrode and a metal oxide electrode. This design allows the device to achieve high energy and power densities by combining the advantages of both components.
Symmetric hybrid supercapacitors, on the other hand, consist of two identical electrodes that can store both charge and energy through both double-layer capacitance and pseudocapacitance. This design allows the device to achieve a high energy density while maintaining a high power density, making it ideal for high-performance applications.
In recent years, several new materials and designs have been developed for hybrid supercapacitors, including graphene-based electrodes, carbon nanotubes, and metal-organic frameworks. These materials offer improved performance and stability compared to traditional materials and have the potential to further enhance the energy storage capabilities of hybrid supercapacitors.
Overall, hybrid supercapacitors represent a promising technology for high-performance energy storage applications. They offer the best of both worlds in terms of high power and energy densities and have the potential to revolutionize the way we store and use energy in the future.
Hybrid Supercapacitors, Pseudocapacitors, and Electrostatic Double-Layer Capacitors (EDLCs) difference in table form
Here’s a table outlining the differences between Hybrid Supercapacitors, Pseudocapacitors, and Electrostatic Double-Layer Capacitors (EDLCs):
Type of Supercapacitor | Working Principle | Energy Storage Mechanism | Charge Storage Mechanism | Advantages | Disadvantages |
---|---|---|---|---|---|
Hybrid Supercapacitors | Combination of EDLC and Pseudocapacitors | Both double-layer and Faradaic mechanisms | Combination of double-layer and Faradaic mechanisms | High energy and power density, longer cycle life than batteries, good stability | Limited scalability, expensive materials |
Pseudocapacitors | Faradaic mechanism | Redox reactions at the electrode surface | Charge transfer between electrolyte and electrode | High specific capacitance, higher energy density than EDLCs, good stability | Limited cycle life, slower charge/discharge rates, dependence on specific electrode materials |
Electrostatic Double-Layer Capacitors (EDLCs) | Double-layer mechanism | Electrical charge separation at the electrode/electrolyte interface | The electrostatic storage of charges at the electrode surface | High power density, fast charge/discharge rates, low cost | Lower energy density than batteries, limited by voltage and dielectric breakdown |
In addition to these three main types, there are other variations of supercapacitors such as asymmetric supercapacitors, which combine an EDLC-like electrode with a battery-like electrode, and microbial supercapacitors, which utilize the metabolic activity of bacteria to generate electrical energy.
Overall, supercapacitors offer a promising solution for energy storage needs in various applications due to their high power density, fast charging and discharging, and long cycle life. With ongoing research and development, new types of supercapacitors are likely to emerge, offering even greater energy storage capabilities and efficiency.
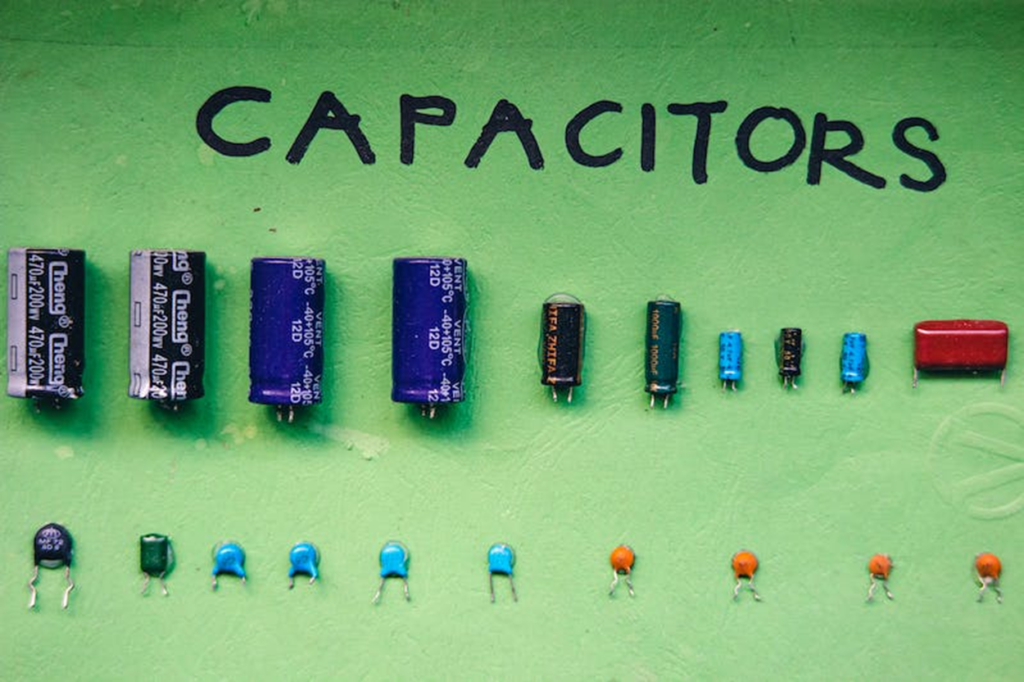
Supercapacitors have a number of practical applications in various fields, some of which are:
- Hybrid and electric vehicles: Supercapacitors are used in conjunction with batteries to provide the high power needed for acceleration and regenerative braking in hybrid and electric vehicles. They can also provide backup power in case of battery failure.
- Renewable energy systems: Supercapacitors can be used to store energy from solar panels or wind turbines, allowing for more efficient use of renewable energy.
- Consumer electronics: Supercapacitors can be used to provide quick bursts of power to devices such as digital cameras, flashlights, and handheld gaming systems.
- Power grid stabilization: Supercapacitors can be used to stabilize the power grid by providing short-term energy storage to compensate for fluctuations in demand or supply.
- Aerospace and defense: Supercapacitors can be used in aerospace and defense applications, such as satellite power systems and weapons systems, due to their ability to withstand extreme temperatures and high shock and vibration.
- Medical devices: Supercapacitors can be used in medical devices such as hearing aids and pacemakers, where they provide reliable and long-lasting power.
- Industrial applications: Supercapacitors can be used in industrial applications such as cranes, forklifts, and elevators, where they provide high power and energy efficiency.
Overall, supercapacitors have numerous applications due to their high power density, fast charge and discharge rates, and long cycle life. Ongoing research is expected to lead to further improvements and applications in the future.