Introduction
Galvanic cells, also known as voltaic cells, are electrochemical devices that convert chemical energy into electrical energy through spontaneous redox reactions. They are widely used in various applications, including batteries and power sources. This article provides a comprehensive overview of galvanic cells, including their principles, construction, operation, and applications.
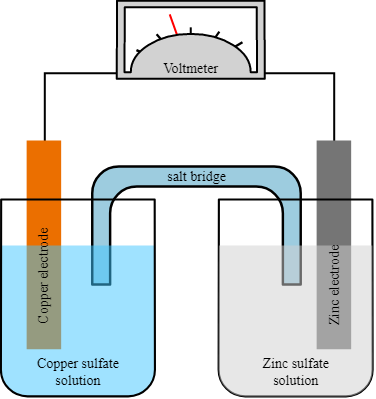
Principles of Galvanic Cells
Galvanic cells operate based on the principles of electrochemistry and redox reactions. They consist of two half-cells, each containing an electrode immersed in an electrolyte solution. The two half-cells are connected by a conductive pathway, allowing the flow of electrons from the anode to the cathode.
The electrical energy produced by a galvanic cell is primarily attributed to the Gibbs free energy of the spontaneous redox reaction occurring within the voltaic cell. Typically, it comprises a pair of half-cells and an intermediary salt bridge. Each half-cell is comprised of a metallic electrode that has been immersed in an electrolytic solution. The two half-cells are externally connected to a voltmeter and a switch through metallic wires. In certain instances, the utilization of a salt bridge may be deemed unnecessary when both electrodes are immersed within a singular electrolytic solution.
- Oxidation Half-Cell: The oxidation half-cell contains the anode, where oxidation occurs. At the anode, the electrode undergoes oxidation, losing electrons and generating metal cations or other oxidized species.
- Reduction Half-Cell: The reduction half-cell contains the cathode, where reduction occurs. At the cathode, the electrode undergoes reduction, gaining electrons and facilitating the reduction of metal cations or other reduced species.
- Electrolyte Solution: The electrolyte solution in each half-cell contains ions that allow the movement of charge and facilitate redox reactions. The composition of the electrolyte is chosen based on the specific requirements of the galvanic cell.
- Salt Bridge: A salt bridge or an ion-permeable membrane is used to connect the two half-cells. It maintains electrical neutrality in each half-cell by allowing the flow of ions while preventing the mixing of the electrolyte solutions.
- Electron Flow: When the two half-cells are connected externally through a conductive wire, electrons flow from the anode to the cathode, creating an electric current. This flow of electrons is driven by the difference in the electrode potentials between the anode and the cathode.
Construction and Components of Galvanic Cells
Galvanic cells consist of several key components that enable their operation and conversion of chemical energy into electrical energy:
- Electrodes: The electrodes in galvanic cells are typically made of conductive materials, such as metals or metal oxides. The choice of electrodes depends on the specific redox reactions involved in the cell and the desired cell performance.
- Electrolyte Solutions: Each half-cell contains an electrolyte solution that facilitates the movement of ions and supports the redox reactions. The electrolyte can be aqueous or non-aqueous, depending on the specific application.
- Separators: Separators are used to physically separate the two half-cells and prevent direct contact between the electrodes. They allow for ion transport while minimizing the possibility of short circuits.
- Conductive Pathway: A conductive pathway, such as a wire or a conductor, connects the two electrodes externally, allowing the flow of electrons from the anode to the cathode. This pathway enables the conversion of chemical energy into electrical energy.
- Salt Bridge or Ion-Permeable Membrane: A salt bridge or an ion-permeable membrane is used to connect the two half-cells. It consists of an electrolyte solution or a porous material that allows the flow of ions while preventing the mixing of the electrolyte solutions. It maintains electrical neutrality in each half-cell.
- External Circuit: The galvanic cell is connected to an external circuit, which may include devices or components that utilize the electrical energy produced by the cell. The external circuit completes the circuit and allows for the flow of electrons, enabling the cell to generate electrical power.
Galvanic Cell Operation
Galvanic cells, also known as voltaic cells, generate electrical energy through spontaneous redox reactions. The operation of a galvanic cell involves the flow of electrons from the anode to the cathode, facilitated by the difference in electrode potentials. Here is a detailed explanation of the steps involved in the operation of a galvanic cell:
- Half-Cell Reactions: In a galvanic cell, there are two half-cell reactions occurring simultaneously at the anode and cathode.
a. Oxidation Half-Cell: At the anode, oxidation takes place. The anode electrode undergoes oxidation, releasing electrons and generating metal cations or other oxidized species. The oxidation reaction is represented as:
Anode: Oxidation → M → M+ + e–
b. Reduction Half-Cell: At the cathode, reduction occurs. The cathode electrode undergoes reduction by gaining electrons and facilitating the reduction of metal cations or other reduced species. The reduction reaction is represented as:
Cathode: Reduction → N+ + e– → N
- Electron Flow: The anode and cathode are connected externally through a conductive pathway, such as a wire or a conductor. The flow of electrons occurs from the anode to the cathode through this external circuit. Electrons are released from the anode during the oxidation reaction and travel through the external circuit toward the cathode.
- Ion Migration: While electrons flow through the external circuit, the galvanic cell maintains electrical neutrality by allowing the migration of ions between the half-cells. This is achieved using a salt bridge or an ion-permeable membrane.
a. Salt Bridge: A salt bridge consists of an electrolyte solution that contains inert ions. It connects the two half-cells, allowing the migration of ions to maintain electrical neutrality. The salt bridge prevents the direct mixing of the electrolyte solutions but enables the movement of ions between the half-cells.
b. Ion-Permeable Membrane: In some cases, an ion-permeable membrane is used instead of a salt bridge. This membrane allows the transport of specific ions while maintaining separation between the electrolyte solutions.
- Reduction and Oxidation Reactions: As the electrons flow through the external circuit from the anode to the cathode, reduction, and oxidation reactions occur at their respective electrodes.
a. Cathode: At the cathode, reduction takes place. Electrons are accepted, facilitating the reduction of metal cations or other reduced species. This reduction reaction occurs due to the presence of the electrons supplied from the anode:
Cathode: Reduction → N+ + e– → N
b. Anode: At the anode, oxidation continues. Electrons are released from the anode, allowing the oxidation of the electrode material and generating metal cations or other oxidized species:
Anode: Oxidation → M → M+ + e–
- Electron Transfer and Electrical Energy Generation: The flow of electrons through the external circuit from the anode to the cathode generates an electric current. This electric current can be utilized to power devices or perform electrical work.
The potential difference between the anode and cathode, known as the cell potential or electromotive force (EMF), is a measure of the driving force for electron flow. The cell potential is determined by the difference in electrode potentials between the two half-cells.
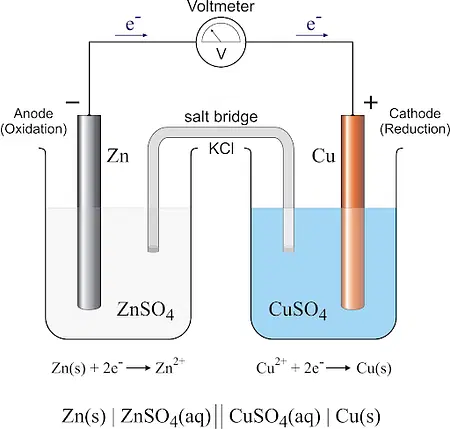
Factors Affecting Galvanic Cell Performance
Several factors can significantly impact the performance of a galvanic cell. Understanding these factors is crucial for optimizing cell efficiency and overall performance. Here are the key factors that influence galvanic cell performance:
- Electrode Materials: The selection of electrode materials is critical as it determines the redox reactions taking place at the electrodes. Different materials have varying redox potentials, which directly affect the cell potential. Electrodes with more positive reduction potentials at the cathode and more negative oxidation potentials at the anode tend to produce higher cell voltages. The choice of materials also affects the stability, reactivity, and conductivity of the electrodes.
- Electrolyte Composition: The composition of the electrolyte solution has a significant impact on the ionic conductivity, ion mobility, and redox reactions in the cell. The choice of electrolyte affects the efficiency of ion transfer between the electrodes, which is crucial for maintaining the electrochemical balance and facilitating redox reactions. The concentration and purity of electrolyte components play a role in the cell’s performance.
- Concentration of Reactants: The concentration of reactants in the electrolyte solutions can influence the reaction rates and, consequently, the cell performance. Higher reactant concentrations generally lead to increased reaction rates, resulting in higher cell currents. However, excessively high concentrations can lead to increased resistive losses and may affect the stability and longevity of the cell.
- Temperature: Temperature has a significant impact on the reaction kinetics and ionic conductivity of the electrolyte. Generally, an increase in temperature accelerates the reaction rates, facilitating faster electron transfer and ion migration. However, excessively high temperatures can lead to increased side reactions, degradation of electrode materials, and reduced cell efficiency. Optimal temperature conditions must be determined for each specific cell design.
- Surface Area and Geometry of Electrodes: The surface area and geometry of the electrodes influence the available reaction sites and the overall current density. Larger electrode surface areas provide more sites for redox reactions and promote higher current outputs. Additionally, the design of the electrode, such as the use of porous structures or catalyst coatings, can enhance reaction kinetics and increase the efficiency of the cell.
- Mass Transport and Stirring: Efficient mass transport of reactants and products to and from the electrodes is critical for maintaining high reaction rates. Stirring the electrolyte solution helps to mitigate concentration polarization and ensures uniform distribution of reactants near the electrode surfaces. Adequate mass transport can be achieved by optimizing flow rates, using stirring mechanisms, or employing porous separators or membranes.
- Catalysts and Coatings: The use of catalysts on electrode surfaces can significantly enhance reaction kinetics and improve overall cell performance. Catalysts facilitate redox reactions, lower activation energies, and increase reaction rates. Various catalyst materials and coatings can be employed, depending on the specific redox reactions involved. Catalysts can also mitigate the effects of impurities or contaminants in the electrolyte solution.
- Cell Design and Configuration: The overall design and configuration of the galvanic cell can impact its performance. Factors such as cell geometry, electrode spacing, current collectors, separators, and the presence of current distribution mechanisms influence the uniformity of the current flow, mass transport, and minimization of resistive losses. Optimal cell design must consider the specific requirements of the application and the desired performance metrics.
Understanding and optimizing these factors are crucial for maximizing the efficiency, longevity, and performance of galvanic cells in various applications, such as batteries, fuel cells, and other electrochemical devices.
Uses of Galvanic Cell
Galvanic cells, also known as voltaic cells, have numerous practical applications due to their ability to convert chemical energy into electrical energy. Here are some of the main uses of galvanic cells:
- Batteries: Galvanic cells serve as the foundation for various types of batteries used in portable electronic devices, vehicles, and renewable energy systems. Batteries power a wide range of devices, including smartphones, laptops, electric vehicles, and grid energy storage systems. Galvanic cells provide a reliable and portable source of electrical energy for these applications.
- Power Sources: Galvanic cells are used as primary power sources in various devices where a long-lasting and compact power supply is required. For example, they are used in hearing aids, pacemakers, remote sensors, and other small electronic devices. Galvanic cells provide a stable and reliable source of power for these applications.
- Backup Power: Galvanic cells are used as backup power sources in critical systems where an uninterrupted power supply is essential. They are commonly used in emergency lighting systems, alarm systems, telecommunications equipment, and uninterruptible power supply (UPS) systems. Galvanic cells ensure that these systems remain operational during power outages or disruptions.
- Automotive Applications: Galvanic cells are used in automotive applications, powering various components such as ignition systems, headlights, and electronic control units. Lead-acid batteries, a type of galvanic cell, are commonly used in automotive vehicles for starting the engine and providing electrical power for the vehicle’s systems.
- Remote and Off-grid Applications: Galvanic cells are utilized in remote and off-grid locations where access to a continuous power supply is limited. They are employed in applications such as remote weather stations, remote sensing devices, agricultural monitoring systems, and off-grid power systems. Galvanic cells provide reliable and self-sustaining power solutions in these isolated environments.
- Corrosion Protection: Galvanic cells are used in cathodic protection systems to prevent corrosion of metal structures, such as pipelines, underground storage tanks, and offshore platforms. By creating a galvanic couple between a sacrificial anode and the structure to be protected, galvanic cells help to direct corrosion away from the structure, prolonging its lifespan and reducing maintenance costs.
- Electroplating and Electrorefining: Galvanic cells are employed in electroplating and electrorefining processes. Electroplating is a technique used to deposit a layer of metal onto a substrate, while electrorefining is used to purify metals. In both processes, a galvanic cell is used to control the flow of electrons and ions, facilitating the desired electrochemical reactions.
- Environmental Monitoring: Galvanic cells are utilized in environmental monitoring systems, such as pH sensors and dissolved oxygen sensors. These sensors rely on galvanic cells to measure the concentration of specific ions or gases in water bodies or air. They play a crucial role in assessing water quality, air pollution, and environmental conditions.
- Educational and Research Purposes: Galvanic cells are extensively used in educational settings to teach the principles of electrochemistry and provide hands-on demonstrations of redox reactions and electrical energy generation. They are also utilized in research laboratories for studying electrochemical processes, developing new materials, and investigating energy storage technologies.
Galvanic cells play a vital role in numerous practical applications, ranging from portable electronics and transportation to corrosion protection and environmental monitoring. Their ability to convert chemical energy into electrical energy makes them an essential component in various industries and everyday technologies.
Top 10 books on Galvanic Cell
Here are ten recommended books that cover the topic of galvanic cells:
- “Electrochemical Systems” by John Newman and Karen E. Thomas-Alyea: This comprehensive textbook covers the principles and applications of electrochemical systems, including galvanic cells.
- “Electrochemical Methods: Fundamentals and Applications” by Allen J. Bard and Larry R. Faulkner: This book provides a detailed introduction to electrochemical methods, including galvanic cells, and covers both theoretical concepts and practical applications.
- “Electrochemical Engineering: Principles” by Vladimir S. Bagotsky: This book focuses on the engineering aspects of electrochemical systems, including galvanic cells, and provides a thorough understanding of electrochemical processes and their applications.
- “Electrochemical Systems” by John Bockris and Amulya K.N. Reddy: This book presents a comprehensive overview of electrochemical systems, including galvanic cells, and covers topics such as electrochemical thermodynamics, kinetics, and transport processes.
- “Modern Electrochemistry” by John O’M. Bockris, Amulya K.N. Reddy, and Maria E. Gamboa-Aldeco: This two-volume set covers various aspects of modern electrochemistry, including galvanic cells, and provides a detailed understanding of electrochemical principles and applications.
- “Principles of Electrochemistry” by P. G. Bruce and A. R. West: This book offers a concise introduction to the principles of electrochemistry, including galvanic cells, and covers topics such as electrode processes, electrochemical thermodynamics, and kinetics.
- “Electrochemical Methods for Neuroscience” by Adrian C. Michael and Laura Borland: This book focuses on the application of electrochemical methods, including galvanic cells, in neuroscience research, covering topics such as biosensors, neurochemical detection, and neuroprosthetics.
- “Electrochemistry” by Carl H. Hamann, Andrew Hamnett, and Wolf Vielstich: This book provides a comprehensive introduction to electrochemistry, including galvanic cells, and covers topics such as electrochemical thermodynamics, kinetics, and modern applications.
- “Handbook of Electrochemistry” edited by Cynthia G. Zoski: This handbook covers a wide range of topics in electrochemistry, including galvanic cells, and provides a valuable resource for researchers and practitioners in the field.
- “Electrochemical Cells: New Advances” edited by Ziyad M. Abu Al-Rub, Eduardo L. Arouca, and Alcínia M. Morais: This book explores recent advances in electrochemical cells, including galvanic cells, and covers topics such as new materials, advanced designs, and emerging applications.
These books provide a wealth of knowledge on galvanic cells and electrochemical systems, covering both fundamental principles and practical applications. They are suitable for students, researchers, and professionals interested in the field of electrochemistry.