Introduction
Redox reactions, also known as oxidation-reduction reactions, are chemical reactions that involve the transfer of electrons between species. These reactions involve the simultaneous occurrence of two half-reactions: oxidation and reduction. In oxidation, a species loses electrons, while in reduction, a species gains electrons.
To understand redox reactions, let’s break down the key concepts:
- Oxidation: Oxidation refers to the loss of electrons by a species. It involves an increase in the oxidation state or a decrease in the number of bonds to hydrogen. In the process, the oxidized species becomes more positive or less negative.
For example Reduction half-reaction:
Cu²⁺ + 2e⁻ → Cu⁰ In this reaction,
copper(II) ions (Cu²⁺) gain two electrons, reducing them to copper (Cu⁰).
- Reduction: Reduction refers to the gain of electrons by a species. It involves a decrease in the oxidation state or an increase in the number of bonds to hydrogen. In the process, the reduced species become more negative or less positive.
For example Oxidation half-reaction:
Zn⁰ → Zn²⁺ + 2e⁻
In this reaction, zinc (Zn⁰) loses two electrons, oxidizing it to zinc(II) ions (Zn²⁺).
- Redox Reaction: A redox reaction occurs when an oxidation reaction and a reduction reaction happen simultaneously. The electrons lost in the oxidation half-reaction are gained in the reduction half-reaction. This transfer of electrons allows for the conservation of charge.
For example, the Redox reaction:
Zn⁰ + Cu²⁺ → Zn²⁺ + Cu⁰
In this reaction, zinc (Zn⁰) is oxidized to zinc(II) ions (Zn²⁺) by losing two electrons, while copper(II) ions (Cu²⁺) are reduced to copper (Cu⁰) by gaining two electrons.
- Oxidizing Agent: The oxidizing agent is a species that causes the oxidation of another species. It accepts electrons and gets reduced in the process. In the above reaction, copper(II) ions (Cu²⁺) act as the oxidizing agent.
- Reducing Agent: The reducing agent is a species that causes the reduction of another species. It donates electrons and gets oxidized in the process. In the above reaction, zinc (Zn⁰) acts as the reducing agent.
- Oxidation Number: The oxidation number, also known as the oxidation state, is a positive or negative number assigned to an atom to indicate its degree of oxidation or reduction in a compound or ion. The change in oxidation number helps identify the species that undergo oxidation or reduction.
Redox reactions are fundamental in various chemical and biological processes. They play a crucial role in energy production, corrosion, combustion, metabolism, and many other chemical transformations. Redox reactions are widely studied and utilized in fields such as chemistry, biochemistry, environmental science, and industry. Understanding redox reactions is essential for comprehending the flow of electrons and energy transfer in chemical systems.
Balancing redox equations involves ensuring that the number of electrons transferred in the oxidation and reduction half-reactions is equal. This is necessary to maintain the conservation of charge and achieve a balanced overall equation. The process of balancing redox equations can be achieved through various methods, including the half-reaction method and the oxidation number method.
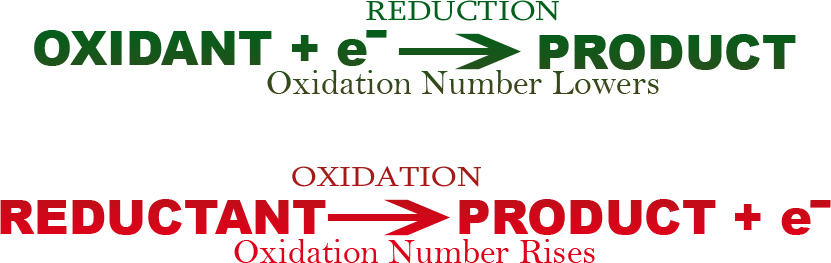
- Half-Reaction Method: The half-reaction method involves separating the overall redox equation into two half-reactions: the oxidation half-reaction and the reduction half-reaction. These half-reactions are balanced individually, ensuring that the number of atoms and the charge is balanced on both sides. Once balanced, the two half-reactions are combined to form a balanced overall equation. The key steps involved in balancing redox equations using the half-reaction method are
- Identify the oxidation and reduction half-reactions in the given equation.
- Balance the atoms in each half-reaction by adjusting coefficients.
- Balance the charges in each half-reaction by adding electrons.
- Multiply the half-reactions by appropriate coefficients to ensure an equal number of electrons transferred in both reactions.
- Combine the balanced half-reactions to form the overall balanced redox equation.
- Oxidation Number Method: The oxidation number method involves assigning oxidation numbers to each element in the reactants and products of the redox equation. By comparing the changes in oxidation numbers, the balanced equation can be determined. The key steps involved in balancing redox equations using the oxidation number method are a. Assign oxidation numbers to all atoms in the reactants and products. b. Identify the elements that undergo a change in oxidation number. c. Determine the number of electrons gained or lost by each atom. d. Balance the electrons gained and lost by multiplying the half-reactions by appropriate coefficients. e. Combine the balanced half-reactions to form the overall balanced redox equation.
Balancing redox equations is crucial for accurately representing chemical reactions involving electron transfer. It allows for the understanding of the stoichiometry of the reactants and products, as well as the calculation of reaction yields and quantities. Balanced redox equations also aid in predicting the products of redox reactions and understanding the underlying principles of chemical transformations involving oxidation and reduction.
Fundamentals of Redox Reactions
- Redox Reactions: Redox reactions, also known as oxidation-reduction reactions, involve the transfer of electrons between species. Oxidation refers to the loss of electrons, while reduction refers to the gain of electrons. These reactions occur simultaneously, and the species that undergoes oxidation is called the reducing agent, while the species that undergoes reduction is called the oxidizing agent.
- Oxidation Number: The concept of oxidation number helps to track the electron transfer in redox reactions. It is a positive or negative number assigned to an atom in a compound or ion to indicate its oxidation state. The oxidation number is based on a set of rules that assign electrons to specific atoms within a compound or ion.
- Oxidation: Oxidation involves an increase in the oxidation number or a loss of electrons. An atom or ion is oxidized when it loses electrons. Common examples of oxidation reactions include the reaction of a metal with oxygen to form a metal oxide or the reaction of a substance with a halogen.
- Reduction: Reduction involves a decrease in the oxidation number or a gain of electrons. An atom or ion is reduced when it gains electrons. Common examples of reduction reactions include the reaction of a metal ion with electrons to form a metal or the reaction of a non-metal with hydrogen to form a hydride.
- Half-Reactions: Redox reactions can be broken down into two half-reactions: the oxidation half-reaction and the reduction half-reaction. The oxidation half-reaction shows the species that loses electrons, while the reduction half-reaction shows the species that gains electrons. By combining the two half-reactions, the overall balanced redox equation can be obtained.
- Reducing Agents and Oxidizing Agents: A reducing agent is a species that donates electrons and undergoes oxidation. It facilitates the reduction of other species. A typical example of a reducing agent is a metal that donates electrons to ions in a redox reaction. An oxidizing agent, on the other hand, is a species that accepts electrons and undergoes reduction. It facilitates the oxidation of another species. A typical example of an oxidizing agent is oxygen, which readily gets electrons in redox reactions.
- Redox Couples: Redox reactions involve the pairing of a reducing agent and an oxidizing agent, forming a redox couple. Each redox couple consists of two species, an oxidized form and a reduced form, that can interconvert through the transfer of electrons. For example, the redox couple Fe²⁺/Fe³⁺ consists of the iron(II) ion (Fe²⁺) and the iron(III) ion (Fe³⁺).
- Redox Potential: Redox potential, also known as oxidation-reduction potential (ORP), is a measure of the tendency of a redox couple to donate or accept electrons. It is a measure of the driving force behind a redox reaction. The redox potential is expressed in volts (V) or millivolts (mV) and can be determined experimentally or calculated based on thermodynamic data.
- Applications of Redox Reactions: Redox reactions have numerous applications in various fields. They are essential in energy storage and conversion devices such as batteries and fuel cells. Redox reactions are also involved in corrosion processes, where metals undergo oxidation in the presence of an oxidizing agent. Additionally, redox reactions play a crucial role in chemical analysis, electroplating, water treatment, and biological processes such as cellular respiration.
Understanding the fundamentals of redox reactions is essential for comprehending the flow of electrons and the transformation of chemical species in various chemical and biological systems. It enables us to predict and control the behavior
Role of Redox Reactions in Electrochemistry
Redox reactions play a fundamental role in electrochemistry, which is the study of the interconversion of chemical and electrical energy. Electrochemistry focuses on the redox processes that occur at the electrode-electrolyte interfaces in electrochemical cells. Here are some key roles of redox reactions in electrochemistry:
- Generation of Electrical Energy: Redox reactions are responsible for the generation of electrical energy in electrochemical cells. In galvanic cells or voltaic cells, a spontaneous redox reaction occurs, leading to the production of electrical energy. The oxidation half-reaction occurs at the anode, where electrons are released, while the reduction half-reaction occurs at the cathode, where electrons are gained. The flow of electrons from the anode to the cathode through an external circuit generates an electric current that can be harnessed for various applications.
- Electrochemical Cells and Batteries: Redox reactions are at the heart of electrochemical cells and batteries. Batteries consist of one or more galvanic cells connected in series or parallel. These cells involve redox reactions that occur between the reactants and products within the cell, allowing for the conversion of chemical energy into electrical energy. The redox reactions that take place during charging and discharging cycles are critical for the functioning of rechargeable batteries.
- Electrolysis: Electrolysis is a process that uses electrical energy to drive a non-spontaneous redox reaction. Through electrolysis, the electrochemical cell facilitates the decomposition of compounds into their constituent ions or elements. This process is widely used in various industrial applications, such as electroplating, electrorefining, water splitting, and the production of chemicals like chlorine and sodium hydroxide.
- Corrosion and Corrosion Prevention: Redox reactions are closely associated with corrosion, which is the deterioration of materials due to chemical reactions to their environment. Corrosion involves the oxidation of a metal, resulting in the formation of metal ions and the reduction of another species, such as oxygen or water. Understanding the redox reactions involved in corrosion processes is crucial for developing strategies to prevent or mitigate corrosion, such as the use of sacrificial anodes or protective coatings.
- Redox Titrations: Redox reactions are widely employed in analytical chemistry, specifically in redox titrations. Redox titrations involve the determination of the concentration of a substance using a redox reaction as the basis of the analysis. These titrations rely on the quantitative transfer of electrons between the analyte and the titrant, allowing for the calculation of the analyte’s concentration.
- Energy Storage and Conversion: Redox reactions are essential for energy storage and conversion devices. Electrochemical systems like fuel cells and supercapacitors utilize redox reactions to store and release electrical energy. Fuel cells involve the oxidation of fuel at the anode and the reduction of an oxidant at the cathode, producing electrical energy. Supercapacitors store energy through the reversible redox reactions occurring at the electrode-electrolyte interface.
Redox reactions are at the core of electrochemistry and are involved in various processes and applications. From the generation of electrical energy in batteries to corrosion prevention and analytical techniques, understanding and manipulating redox reactions are essential for advancing electrochemical technologies and their practical applications.
Top 10 books on Redox reactions
Here are ten recommended books on redox reactions that cover various aspects of the topic:
- “Electrochemical Methods: Fundamentals and Applications” by Allen J. Bard and Larry R. Faulkner – This comprehensive textbook provides an in-depth understanding of electrochemical methods, including redox reactions, their principles, and their applications in analytical chemistry and electrochemical energy storage.
- “Modern Electrochemistry 2A: Fundamentals of Electrodics” by John O’M. Bockris and Amulya K.N. Reddy – This book covers the fundamentals of electrochemistry, including redox reactions, electrode kinetics, and the application of electrochemical methods in various fields.
- “Electrochemical Reactions and Mechanisms in Organic Chemistry” by J. M. Saveant – Focusing on the role of redox reactions in organic chemistry, this book explores the mechanisms and applications of electrochemical reactions in organic synthesis and electroorganic chemistry.
- “Principles of Electrochemistry” by P. Horsman and M. M. Pintar – This textbook provides a comprehensive introduction to the principles of electrochemistry, including redox reactions, electrochemical cells, and the fundamentals of electrode processes.
- “Redox Biochemistry” by Joseph J. Stephanos and Richard L. Edelstein – This book focuses on the role of redox reactions in biological systems, covering topics such as oxidative stress, antioxidant mechanisms, and redox signaling in cellular processes.
- “Electrochemistry” by Carl H. Hamann, Andrew Hamnett, and Wolf Vielstich – This textbook offers a comprehensive overview of electrochemistry, including redox reactions, electrochemical cells, and applications in energy conversion and storage.
- “Electrochemical Engineering: Principles” by Thomas F. Fuller, John Newman, and Steven J. Benson – This book explores the principles of electrochemical engineering, covering topics such as redox reactions, electrochemical kinetics, and the design of electrochemical devices and processes.
- “Redox: Fundamentals, Processes and Applications” edited by Mieczyslaw Lapkowski – This book provides a collection of chapters written by leading experts in the field, covering various aspects of redox reactions, including theoretical foundations, experimental techniques, and applications in energy, environmental, and biological systems.
- “Electroanalytical Chemistry: A Series of Advances” edited by Allen J. Bard – This series of books offers a collection of advanced topics in electroanalytical chemistry, including redox reactions, electrochemical sensors, and the application of electroanalytical techniques in various fields.
- “Electrode Dynamics” by Piotr Skeldon – This book focuses on the dynamics of electrochemical reactions at solid electrodes, covering topics such as redox reactions, interfacial processes, and the kinetics of electrode reactions.
These books provide a comprehensive understanding of redox reactions, their principles, and their applications in various fields of chemistry, electrochemistry, and biochemistry. They cater to different levels of expertise, ranging from introductory textbooks to advanced research monographs, allowing readers to delve into the fascinating world of redox chemistry.
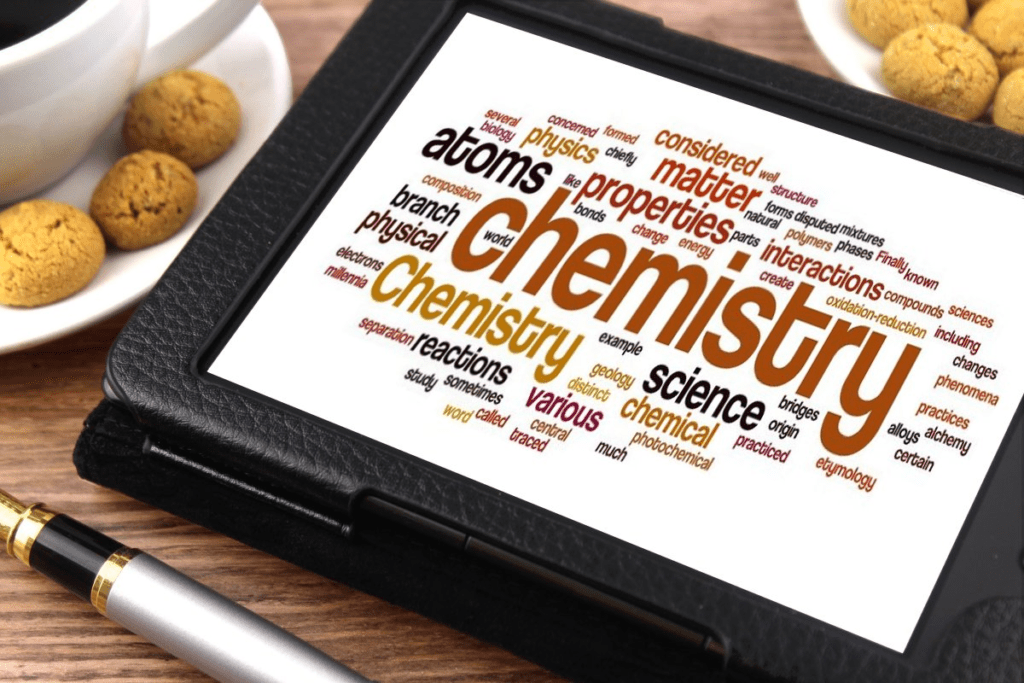