Superconducting Magnetic Energy Storage
Introduction
Superconducting magnetic energy storage (SMES) is a type of energy storage system that uses superconducting coils to store energy in the form of a magnetic field. SMES systems can store large amounts of energy for long periods of time with very little energy loss, making them ideal for use in applications such as power grid stabilization and backup power for critical systems.
The basic principle behind SMES is that electrical current flowing through a superconducting coil creates a magnetic field, which can then be used to store energy. The superconducting coil is typically made from a material such as niobium-titanium, which has zero electrical resistance when cooled to very low temperatures.
To charge an SMES system, electrical energy is used to create a magnetic field in the superconducting coil. When the system needs to discharge, the magnetic field is used to generate electrical energy, which can be used to power devices or feed back into the power grid.
SMES systems have several advantages over other types of energy storage systems. They have high energy density, which means they can store a large amount of energy in a relatively small space. They also have high power density, which means they can deliver a large amount of power quickly. Additionally, SMES systems have very high efficiency, with very little energy lost as heat during the charging and discharging cycles.
However, SMES systems also have some limitations. They require very low temperatures to operate, typically below 10 Kelvin (-263 degrees Celsius), which can be difficult and expensive to achieve. They also have relatively high initial costs, although these costs may be offset by their long lifespan and high efficiency.
Fundamental of superconducting Magnetic Energy Storage system:
Superconducting Magnetic Energy Storage (SMES) is a type of energy storage technology that uses superconducting coils to store electrical energy in the form of a magnetic field. SMES systems are based on the principle of superconductivity, which is a phenomenon where certain materials exhibit zero electrical resistance and complete expulsion of magnetic fields when cooled to very low temperatures.
The basic components of an SMES system include a superconducting coil, a power conditioning system, and a cryogenic cooling system. The superconducting coil is made of a superconducting material such as niobium-titanium or niobium-tin, which is cooled to a very low temperature using a cryogenic cooling system such as liquid helium.
To charge the SMES system, a current is passed through the superconducting coil, which generates a strong magnetic field. This magnetic field is stored in the coil until the energy is needed, at which point the magnetic field is used to generate an electrical current through the coil, which can be used to power devices or feed back into the power grid.
SMES systems have several advantages over other energy storage technologies, including high energy density, high power density, and high efficiency. SMES systems can store a large amount of energy in a relatively small space and can deliver that energy quickly with very little energy loss. Additionally, SMES systems have a long lifespan, with little degradation over time.
However, SMES systems also have some limitations, including the requirement for very low temperatures, which can be difficult and expensive to achieve. Additionally, SMES systems have relatively high initial costs compared to other energy storage technologies. Despite these limitations, SMES systems have shown promise in applications such as power grid stabilization, renewable energy integration, and backup power for critical systems.
Benefits of superconducting Magnetic Energy Storage:
Superconducting Magnetic Energy Storage (SMES) offers several benefits as an energy storage technology, including:
- High energy density: SMES systems can store a large amount of energy in a relatively small space, making them ideal for applications where space is limited.
- High power density: SMES systems can deliver a large amount of power quickly, making them suitable for applications that require high power output.
- High efficiency: SMES systems have very little energy loss during the charging and discharging cycles, which means that they are highly efficient.
- Long lifespan: SMES systems have a long lifespan and can last for several decades with minimal maintenance.
- Fast response time: SMES systems can respond quickly to changes in demand, making them ideal for applications such as grid stabilization and backup power.
- Environmentally friendly: SMES systems do not emit any pollutants or greenhouse gases, making them a clean energy storage technology.
- Reliable: SMES systems are highly reliable and can provide an uninterrupted power supply for critical systems.
- Low maintenance: SMES systems require minimal maintenance, which reduces operational costs and downtime.
- Modular: SMES systems are modular, which means that they can be scaled up or down to meet changing energy storage needs.
Overall, the benefits of SMES systems make them a promising energy storage technology for a wide range of applications, including renewable energy integration, grid stabilization, and backup power for critical systems.
Limitation of superconducting Magnetic Energy Storage:
Superconducting Magnetic Energy Storage (SMES) technology has several limitations, including:
- High cost: SMES systems are relatively expensive to manufacture and operate, mainly due to the cost of the superconducting materials used and the cryogenic cooling systems required to maintain the low temperatures necessary for operation.
- Limited energy storage capacity: SMES systems typically have limited energy storage capacity compared to other energy storage technologies, such as pumped hydro storage and battery storage.
- Complex design: SMES systems require complex and sophisticated design to maintain the necessary low temperatures and ensure optimal operation, which can increase manufacturing and maintenance costs.
- Low operating temperature: SMES systems require extremely low temperatures (typically below 10 Kelvin or -263 degrees Celsius) to operate, which can make their deployment challenging and expensive.
- Safety concerns: SMES systems require careful handling of cryogenic materials, which poses safety risks and requires specialized personnel.
- Space requirements: SMES systems require a significant amount of space to accommodate the superconducting coil and associated equipment, which can limit their deployment in some applications.
Despite these limitations, SMES technology has several advantages, such as high efficiency, high power density, and long lifespan, that make it a promising energy storage technology for certain applications. Advances in materials science and cryogenic engineering may help overcome some of these limitations in the future.
Future of superconducting Magnetic Energy Storage:
The future of Superconducting Magnetic Energy Storage (SMES) technology looks promising, as researchers and engineers continue to explore and develop new applications and improvements to the technology. Some potential future developments for SMES technology include:
- Improved materials: Advances in materials science could lead to the development of new superconducting materials that are more efficient and cost-effective than the current materials used in SMES systems. For example, researchers are exploring the use of high-temperature superconducting materials, which would eliminate the need for expensive cryogenic cooling systems.
- Increased energy storage capacity: Researchers are working on improving the energy storage capacity of SMES systems, potentially by increasing the size of the superconducting coil or using more advanced control systems to optimize energy storage.
- Cost reduction: Advances in manufacturing processes and materials could help to reduce the cost of SMES systems, making them more competitive with other energy storage technologies.
- Expansion of applications: SMES systems could potentially be used in a wider range of applications, such as transportation, energy transmission, and space exploration.
- Integration with renewable energy: SMES systems could be used to store energy generated from renewable sources such as wind and solar, helping to smooth out fluctuations in energy supply and demand and increase the reliability of renewable energy systems.
- Microgrid applications: SMES systems could be used in microgrids to provide backup power and improve grid stability in remote areas or areas with unreliable power grids.
Overall, the future of SMES technology looks promising, with the potential for improved efficiency, increased energy storage capacity, and new applications. As the demand for reliable and sustainable energy storage solutions continues to grow, SMES technology is likely to play an increasingly important role in meeting this demand.
Comparision of Superconducting Magnetic Energy Storage with other energy storage
Here’s a comparison table of Superconducting Magnetic Energy Storage (SMES) with other common energy storage technologies:
Energy Storage Technology | Advantages | Disadvantages | Applications |
---|---|---|---|
SMES | High energy density, fast response time, long cycle life, low maintenance, no emissions or pollution, high efficiency | High cost, requires cryogenic cooling, limited energy storage capacity | Power grid stability, load balancing, backup power for critical infrastructure, aerospace and defense applications |
Lithium-ion Batteries | High energy density, low self-discharge, scalable, mature technology | Limited cycle life, potential for thermal runaway, safety concerns, limited power output | Electric vehicles, residential and commercial energy storage, backup power |
Pumped Hydro Storage | Large-scale energy storage, long cycle life, low cost, proven technology | Geographically limited, requires significant infrastructure, limited energy density, long response time | Power grid stability, load balancing, energy storage for renewable sources |
Compressed Air Energy Storage | Large-scale energy storage, long cycle life, low cost, proven technology | Geographically limited, low energy density, limited efficiency, long response time | Power grid stability, load balancing, energy storage for renewable sources |
Flywheels | Fast response time, long cycle life, scalable, no emissions or pollution, high efficiency | Limited energy storage capacity, high cost, requires precise engineering and maintenance | Power grid stability, load balancing, backup power |
Capacitors | Fast response time, long cycle life, low maintenance, high efficiency | Limited energy storage capacity, relatively low energy density | High power output applications, electric vehicles, backup power |
It’s worth noting that the choice of energy storage technology depends on the specific application and the required balance of energy density, power output, response time, cycle life, and cost. Different energy storage technologies may be more suitable for different use cases, and a combination of multiple technologies may be used to optimize energy storage for a given application.
List of top 10 books on Superconducting Magnetic Energy Storage
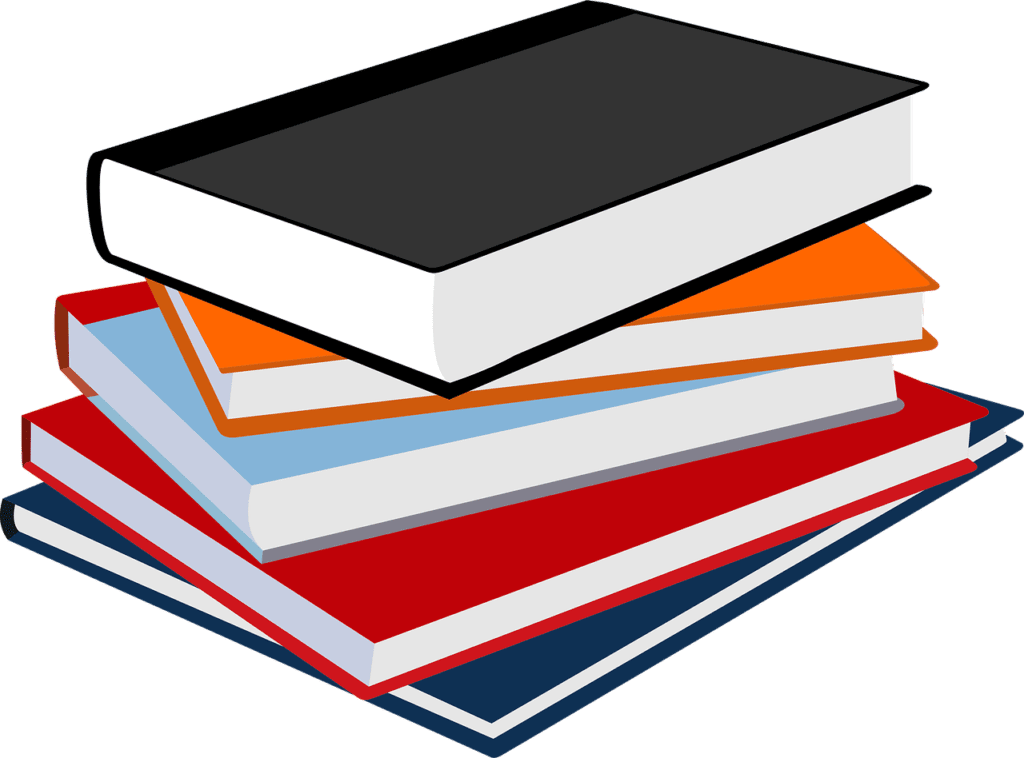
Here are top 10 books on superconducting Magnetic Energy Storage
- “Superconducting Magnetic Energy Storage (SMES): Concepts, Systems, and Applications” by Ronald W. Boom and Ram Adapa
- “Superconducting Energy Storage Systems: Architecture and Technology for Grid-Scale Applications” by Ziad Melhem, Paul Konopka, and Jack Kessler
- “Superconducting Magnetic Energy Storage (SMES) Handbook” by Carol A. Stripling
- “Superconducting Magnetic Energy Storage (SMES) Power Systems” by Yeong-Jun Kim and Seong-Joo Lim
- “Superconducting Magnetic Energy Storage: Fundamentals and Applications” edited by Tarek Elsayed and Ahmed Massoud
- “Superconductivity: A Very Short Introduction” by Stephen Blundell
- “Superconductivity: An Introduction” by Reinhold Kleiner and Werner Buckel
- “Introduction to Superconductivity” by Michael Tinkham
- “Superconductivity: Fundamentals and Applications” by Matthias Eschrig
- “Superconducting Materials and Applications: Advanced Texts in Physics” by Teruo Matsushita and Takanobu Kiss.