A battery can be recharged
Introduction
Yes, a battery can be recharged. Rechargeable batteries are designed to be used multiple times, and they can be recharged using a compatible charger. When a rechargeable battery is depleted, the chemical reactions that produce the electrical energy have reached equilibrium, and the battery must be recharged to restore its energy storage capacity.
The recharging process involves reversing the chemical reactions that occurred during discharge. This is done by applying an external electrical current to the battery, which forces the chemical reactions to occur in the opposite direction. The energy from the electrical current is stored in the battery, restoring its energy storage capacity.
Different types of rechargeable batteries have different charging requirements. For example, nickel-cadmium (Ni-Cd) batteries should be charged at a constant current and voltage to prevent overcharging, which can damage the battery and reduce its lifespan. Lithium-ion (Li-ion) batteries, on the other hand, require a more complex charging algorithm that involves monitoring the battery’s voltage and temperature to ensure safe and efficient charging.
It is important to use the correct charger for a rechargeable battery to prevent damage to the battery and ensure safe charging. Using a charger that is not compatible with the battery can result in overcharging, overheating, and other safety hazards.
Overall, rechargeable batteries offer several advantages over disposable batteries, including reduced waste, cost savings, and convenience. They can be recharged many times, making them a more sustainable and environmentally friendly choice for powering electronic devices.
Rechargeable batteries are also sometimes referred to as secondary batteries. It is linked to a load, which is used to refer to any device or system that uses power, and it stores energy. A chemical process helps drain the battery whenever the load needs power. This happens whenever the battery is being used. This reaction may be turned around to provide a fresh charge to the battery.
In essence, these batteries may be used several times, which makes them one of the most cost-effective methods of storage due to the fact that multiple uses can be derived from a single purchase. The lead-acid battery, the lithium-ion battery, the nickel-cadmium battery, and the nickel-metal hydride battery are all types of rechargeable batteries.
It is common practice in data centers, telecom sites, and other types of industrial facilities to employ lead-acid and lithium-ion rechargeable batteries in their uninterruptible power supply (UPS), which serves as a backup power source in the event that the primary power supply fails.
Battery with Flow
One kind of rechargeable battery is the flow battery, which differs in that it is more modular. Flow batteries, on the other hand, have a very low self-discharge quotient, which indicates that a greater amount of energy is preserved compared to the latter.
These batteries are often used by electric cars because of their rapid charging and discharging capabilities as well as their high energy demand. In addition to this, they are used in Original Equipment Manufacturers (OEMs), as well as in grid energy storage, both of which need a significant amount of energy capacity. As a consequence of this, there must be a reliable source of electricity that is not interrupted.
These many methods of energy storage are capable of working well on their own, but new firms of the next generation are heading toward integrated solutions that combine battery storage with monitoring technologies.
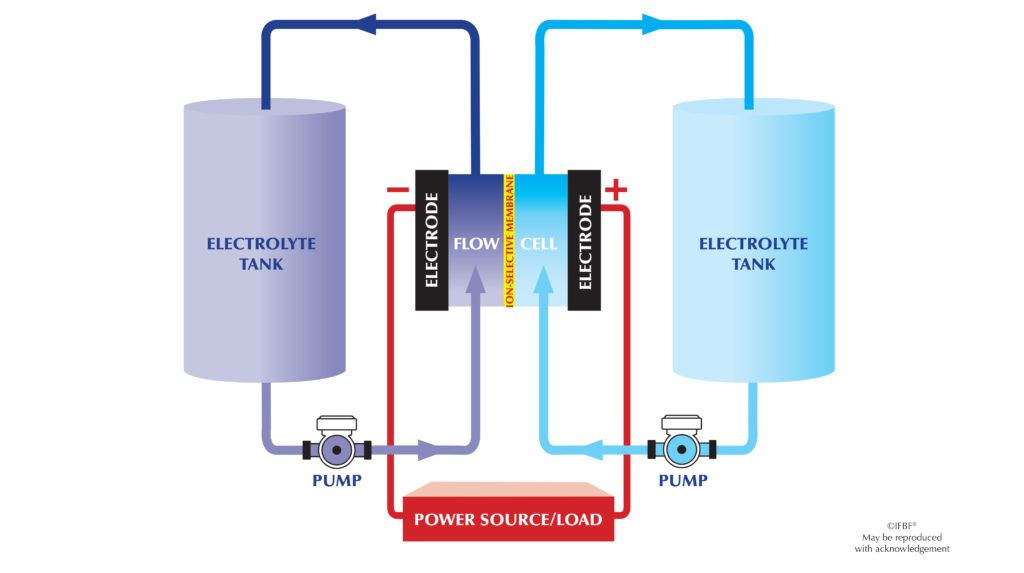
A battery with the flow, also known as a flow battery, is a type of rechargeable battery that uses two different fluids, called electrolytes, which flow through separate chambers of the battery. The electrolytes are stored in large tanks outside the battery, allowing for easy replacement and recharging.
The process of energy storage in a flow battery works as follows:
- During charging, the two electrolytes are pumped into the battery, where they react and store electrical energy in the form of chemical bonds.
- During discharging, the electrolytes flow through a membrane that separates the two chambers of the battery. As they flow, they react and release electrical energy, which can be used to power devices.
- The amount of energy stored in the battery depends on the size of the tanks and the flow rate of the electrolytes.
Flow batteries have several advantages over other types of batteries. For example:
- They have a high energy capacity and can store large amounts of energy for long periods of time.
- They can be easily scaled up or down to meet different energy demands.
- They have a long lifespan, with some flow batteries lasting up to 20 years.
- They are environmentally friendly, with low emissions and the potential to use renewable energy sources as the input energy for charging.
However, there are also some challenges associated with flow batteries, such as the high cost of the membrane and the need for regular maintenance to prevent clogging and leaks.
System for the Monitoring of Batteries
The technology for monitoring batteries works in conjunction with storage systems to improve the latter’s functionalities. These features allow for remote monitoring of the performance of the battery as well as optimization of that performance with the assistance of analytics and intelligence systems.
These have the potential to assist businesses in proactively predicting potential catastrophes and risks, adjusting supply and demand on the fly based on real-time data, and providing further insights into the whole system.
Energy may be stored using the electric and magnetic characteristics of various pieces of equipment, such as capacitors, supercapacitors, and superconducting magnets, in a process known as electromagnetic storage.
Battery monitoring systems are used to monitor the performance, health, and state of charge of batteries in real time. These systems are commonly used in industrial and automotive applications where batteries are critical to the operation of the equipment.
A battery monitoring system typically consists of several components, including sensors, a data acquisition unit, a processing unit, and a user interface. The sensors are used to measure parameters such as battery voltage, current, temperature, and state of charge. The data acquisition unit collects the data from the sensors and sends it to the processing unit for analysis. The processing unit then analyzes the data and provides information on the battery’s performance, health, and state of charge. The user interface displays the information to the user in an easy-to-understand format.
The monitoring system can provide the following information:
- Battery voltage: The voltage of the battery can be measured to determine if it is charged or discharged.
- Battery current: The current flowing into or out of the battery can be measured to determine if the battery is being charged or discharged.
- Temperature: The temperature of the battery can be measured to determine if it is within safe operating limits.
- State of charge: The state of charge of the battery can be measured to determine how much energy is left in the battery.
- State of health: The state of health of the battery can be measured to determine if it is functioning properly or if it needs to be replaced.
A battery monitoring system can help prevent battery failure, extend battery life, and optimize battery performance. By monitoring the battery in real time, the system can detect and diagnose problems early, allowing for proactive maintenance and reducing the risk of unplanned downtime.
Capacitor
Two plates are used in the construction of a capacitor, and an insulator is placed in between them. The energy is stored in the form of electrostatic charges through this process. In most cases, they will be linked to some kind of charging circuit. When it is detached, the device saves energy so that it may be utilized later, such as when the batteries are replaced. As a result, it contributes to the provision of energy, which helps to sustain the power supply while the battery is being changed.
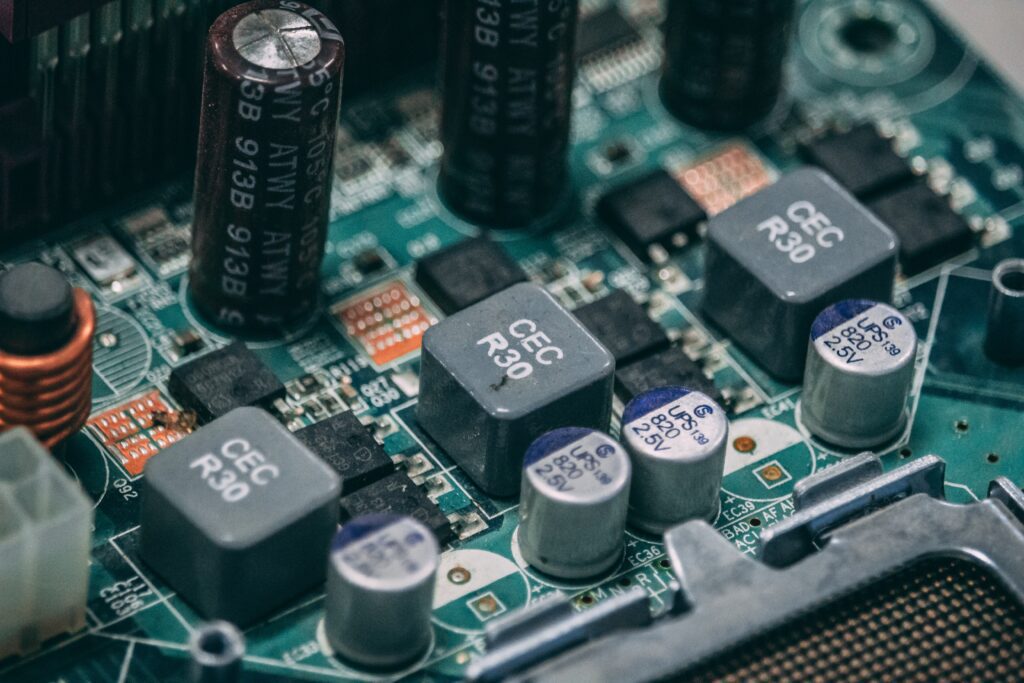
A capacitor is an electrical component that stores electrical energy in an electric field. It is made up of two metal plates separated by a dielectric material, such as air or plastic. When a voltage is applied across the plates, an electric field is created between them, causing the plates to store electrical charge.
The capacitance of a capacitor is a measure of its ability to store electrical charge, and is determined by the size of the plates, the distance between them, and the dielectric material between them. Capacitance is measured in farads (F), with a one farad capacitor being able to store one coulomb of electrical charge at one volt.
Capacitors have a wide range of applications in electronic circuits, including:
- Energy storage: Capacitors can be used to store electrical energy in circuits where a small amount of energy needs to be released quickly.
- Filtering: Capacitors can be used to smooth out fluctuations in voltage and current in electronic circuits.
- Timing: Capacitors can be used in conjunction with resistors to create timing circuits, such as in oscillators.
- Coupling: Capacitors can be used to connect two circuits while blocking DC voltage, allowing for AC signals to be transmitted.
- Power factor correction: Capacitors can be used to improve the power factor of electrical systems by storing and releasing energy to counteract reactive power.
- Motor starting: Capacitors can be used to start single-phase AC motors by creating a phase shift between the start winding and the main winding.
There are many different types of capacitors, including electrolytic capacitors, ceramic capacitors, tantalum capacitors, and film capacitors, each with their own unique properties and applications.
Supercapacitor
The difference between capacitors and rechargeable batteries is closed by a device called a supercapacitor. In comparison to capacitors, it has a greater energy density and can complete charging and discharging cycles in a shorter amount of time.
Even though these are some of the most important forms of storage, there are many more, such as storage for fossil fuels, thermal storage, biological storage, and chemical storage. These have varying degrees of significance across a variety of industries. Therefore, let’s take a look at why they are so vital as well as the advantages they provide.
Supercapacitors, also known as ultracapacitors or electrochemical capacitors, are energy storage devices that store electrical energy in an electrostatic field rather than in a chemical reaction like batteries. They have several advantages over traditional batteries, such as fast charge and discharge rates, high power density, and long cycle life.
Supercapacitors consist of two electrodes, separated by an electrolyte, and a separator that prevents direct contact between the electrodes. The electrodes are typically made of activated carbon, which provides a large surface area for the electrolyte to interact with. The electrolyte can be a liquid or a gel, and it provides ions that move between the two electrodes during charging and discharging, allowing for energy storage.
During charging, ions from the electrolyte accumulate on the surface of the electrodes, creating an electrostatic field. The amount of energy that can be stored in a supercapacitor is proportional to the surface area of the electrodes and the distance between them. This means that supercapacitors can store more energy than traditional capacitors, but less energy than batteries.
Supercapacitors can be charged and discharged much faster than batteries, which makes them ideal for applications that require high power output. They also have a longer cycle life than batteries, which means they can be charged and discharged more times before they start to degrade.
One of the main disadvantages of supercapacitors is their low energy density, which means they can store less energy per unit of volume or weight than batteries. This limits their use in applications that require high energy storage, such as electric vehicles. However, research is ongoing to develop new materials and designs that could increase the energy density of supercapacitors.
Another disadvantage of supercapacitors is their voltage drop during discharge, which means that their output voltage decreases as they discharge. This can be problematic in some applications that require a constant output voltage.
Despite these limitations, supercapacitors have many potential applications, including in renewable energy systems, transportation, and electronics. In renewable energy systems, supercapacitors can be used to smooth out the fluctuations in power output from renewable sources, such as wind and solar power. In transportation, supercapacitors can be used to provide short bursts of high power to electric vehicles, improving their performance and range. In electronics, supercapacitors can be used to power devices that require high power output, such as cameras and flashlights.
In conclusion, supercapacitors are energy storage devices that offer several advantages over traditional batteries, such as fast charge and discharge rates, high power density, and long cycle life. While they have lower energy density than batteries, they have many potential applications in renewable energy systems, transportation, and electronics. Research is ongoing to develop new materials and designs that could increase their energy density and expand their use in a variety of applications.